Volume 7 - Year 2019 - Pages 16-23
DOI: 10.11159/ijtan.2019.003
Effect of Preparation Method on the Performance for PROX of Gold Catalysts on Alumina Supported Y-Doped Ceria
Lyuba Ilieva1, Petya Petrova1, Giuseppe Pantaleo2, Rodolfo Zanella3, Zbigniew Kaszkur4, Leonarda F. Liotta2, Anna Maria Venezia2, Tatyana Tabakova1
1Bulgarian Academy of Sciences, Institute of Catalysis
Acad. G. Bonchev str., bl.11, Sofia 1113, Bulgaria
luilieva@ic.bas.bg; petia@ic.bas.bg; tabakova@ic.bas.bg
2CNR, Institute of Nanostructured Materials (ISMN)
Via Ugo La Malfa 153, Palermo I - 90146, Italy
giuseppe-pantaleo@cnr.it; leonardafrancesca.liotta@cnr.it; venezia@pa.ismn.cnr.it
3Universidad Nacional Autónoma de México, Instituto de Ciencias Aplicadas y Tecnología
México 04510, Mexico
rodolfo.zanella@icat.unam.mx
4Polish Academy of Sciences, Institute of Physical Chemistry
Kasprzaka str. 44/52, Warsaw 01-224, Poland
zkaszkur@ichf.edu.pl
Abstract - Gold catalysts on CeO2/Al2O3 and Y-doped CeO2/Al2O3 supports (20 wt.% CeO2 and 1 wt.% Y2O3) were synthesized. The mixed oxide supports were prepared by impregnation (IM) or by mechanical mixing (MM). The samples were characterised by BET, XRD and HRTEM/HAADF measurements. The catalytic properties in the preferential CO oxidation in hydrogen rich stream (PROX) using gas feed composition: 60% H2 + 1% CO + 1% O2 (He as balance) were estimated. The effect of supports preparation on the gold loading and its dispersion, supports features and catalytic performance was commented. The positive effect of yttrium for catalysts stability was evidenced during long run tests in PROX with CO2 and water addition to gas stream. The obtained results could be useful for the design of catalysts for CO-free hydrogen production with good PROX performance at reasonable price.
Keywords: Nanosized gold catalysts, alumina supported Y-doped ceria, PROX.
© Copyright 2019 Authors - This is an Open Access article published under the Creative Commons Attribution License terms. Unrestricted use, distribution, and reproduction in any medium are permitted, provided the original work is properly cited.
Date Received: 2019-09-16
Date Accepted: 2019-10-21
Date Published: 2019-11-06
1. Introduction
The proton exchange membrane fuel cells (PEMFC) require hydrogen purification from carbon monoxide up to the levels below 10 ppm for Pt anodes and below 100 ppm for CO-tolerant alloy anodes. The preferential CO oxidation in H2-rich stream (PROX) is a cheap and efficient way to decrease the CO concentration in the stream after the reforming process and the subsequent water gas shift reaction. The effective PROX catalysts must meet some specific requirements: i) high CO oxidation activity but low activity for the unwanted H2 oxidation in the region of relatively low PEMFC operating temperatures between 80 – 120 oC; ii) reduced harmful effect of water and especially of CO2, usually present in the reformate stream. Regarding the latter the improved resistance to CO2 is characteristic for acidic as compared to the basic supports [1]. The established by Haruta and co-workers [2] extraordinary activity in CO oxidation over supported highly dispersed gold nanoparticles contributed to an increased level of interest for catalysis by gold. Gold catalysts were objects of many studies related to PROX because at low temperature higher rate of CO oxidation than that of H2 oxidation was observed [3,4]. It is generally agreed that the catalytic activity of gold-based catalysts strongly depends on the gold particles dispersion. At the same time, the support nature and features, preparation method and catalyst pretreatment are also important factors [1,5]. Ceria has received a special attention among the appropriate supports for gold catalysts because of the well-known enhanced redox transfer Ce4+↔Ce3+ and the ability to promote gold dispersion by the presence of surface oxygen vacancies as nucleation sites for small particles [6]. Concerning PROX it has been proposed that the predominant mechanism over gold/ceria catalysts is the Mars–van Krevelen mechanism with the ceria lattice oxygen involvement [7]. Carabineiro et al. evidenced that there is a correlation between CO oxidation activity and lattice oxygen supply by ceria [8]. The modification of ceria by appropriate metal dopants could increase the oxygen vacancies creation leading to higher number of surface sites for gold deposition as well as improving the support redox properties. A relatively new approach for development of catalytically efficient but also cost-effective gold/ceria catalysts was proposed by Odriozola and coauthors [9-12]. This new strategy motivated our very recent study of PROX over gold on CeO2/Al2O3 and Y-doped CeO2/Al2O3 supports. The ceria amount was 10, 20 or 30 wt.% and the mixed oxide supports were prepared by impregnation [13] or by mechanical mixing [14]. The addition of yttrium was 1 wt.% Y2O3 on the bases of promising results previously obtained in PROX over gold deposited on Y-modified ceria supports [15]. In agreement with literature data [16], the optimal ceria amount established with respect to CO conversion and selectivity was 20 wt.%.
In the present study the gold catalysts for PROX on CeO2/Al2O3 and Y-doped CeO2/Al2O3 (1 wt.% Y2O3) supports with 20 wt.% ceria deposition by impregnation or mechanical mixing are compared. The effects of preparation method on: i) gold loading and dispersion; ii) supports features and iii) catalytic activity, selectivity and stability in PROX are discussed.
2. Experimental
2. 1. Samples preparation
The alumina supported ceria and Y-modified ceria samples were prepared by two different methods: wet impregnation (IM) and mechanical mixing (MM). The amount of ceria was 20 wt.%. The Y-doping was 1 wt.% Y2O3 with respect to the ceria content. Using the first method, γ-alumina (Sasol) was impregnated with aqueous solution of Ce(NO3)3.6H2O via vigorous stirring for 4 h at room temperature and thereafter the complete water removal was performed at 70 oC using rotary evaporator. The precursor was calcined in air for 2 h at 400 oC. The same impregnation procedure was repeated for the synthesis of the Y-doped ceria adding aqueous solution of Y(NO3)3.6H2O. For the MM method of preparation, cerium hydroxide was previously synthesized by precipitation of Ce(NO3)3·6H2O with K2CO3 at 60 oC and constant pH = 9.0. The precipitate was aged at the same temperature for 1 h, filtered, washed until the removal of NO3−ions and dried in vacuum at 80 oC. Then, alumina and the calculated amount of cerium hydroxide were mechanically mixed in a mortar for for 30 min and then calcined at 400 oC for 2 h. The Y-containing support was prepared by the same procedure of mechanical mixing with addition of the calculated amount of Y2O3. The thermal treatment was carried out in air at 400 oC for 2 h.
Gold (3 wt.%) was loaded by deposition-precipitation method at pH 7.0 and 60 oC following the procedure already reported in Ref. [15]. The gold catalysts were denoted as Au20CeAlIM and Au20CeAlMM as well as AuY20CeAlIM and AuY20CeAlMM. Gold on ceria and gold on alumina, designated as AuCe and AuAl respectively, were also synthesized for comparison.
2. 2. Samples characterization
The estimation of actual gold loading (±0.05 wt.%) was performed by X-ray fluorescence (XRF) analysis using energy dispersive MiniPal4 XRF desktop spectrometer equipped with SDD detector of 145 eV resolution with Rh anode tube.
The BET equation was applied for determination of the specific surface areas of the catalysts (SBET) using Quantachrome Instruments NOVA 1200e (USA) at −196 oC in the pressure range 0.05 – 0.30 p/po. The mean pore size diameter was calculated by BJH method applied to the desorption branch. The accuracy of each measured point was 2%. The samples were outgassed under vacuum at 200 oC for 90 min prior to the measurements.
The powder X-ray diffraction (XRD) patterns were obtained by means of D5000 diffractometer from Bruker AXS. More detailed description of the XRD measurements and the analysis are given in Ref. [13].
JEM 2010 FasTem analytical microscope provided with a Z-contrast annular detector was used to obtain high resolution transmission electron microscopy (HRTEM) and high angle annular dark field (HAADF) observations of the studied gold catalysts. The gold particle size histograms were established by the measurements of more than 700 particles on the basis of the corresponding Z-contrast observations. The formula DAu = ∑niDi/∑ni (where ni is the number of particles with diameter Di) was applied for calculation of the mean size of gold particles (DAu) The standard error related to the accuracy of the values reported in both the average diameter and the histogram distributions was in the frame 0.03 – 0.08.
2. 3. Catalytic activity, selectivity and stability in PROX
The degree of CO conversion and the selectivity toward CO2 were evaluated in the temperature range 30–180 oC by means of ABB infrared analyser for CO and CO2 detection and ABB paramagnetic Magnos206 for O2 analysing. The sample of 0.05 g was situated in a U-shaped quartz glass reactor, equipped with a temperature programmed controller. The gas feed was containing 60% H2 + 1% CO + 1% O2 and He as balance. The used total flow rate was 50 mL min-1 (WHSV of 60 000 mL g−1 h-1). Catalytic tests at realistic PROX conditions were also performed adding to the gas feed 10% CO2 and 10% H2O. The H2O addition was realised using gas mixture bubbling in water at a temperature (46 °C) giving equilibrium of 10% H2O with the gas phase. Long term stability tests at these conditions and at 100 oC (the temperature of the highest CO conversion) were carried for 24 h. On the basis of preliminary tests following catalyst pretreatment in oxidizing or reductive atmosphere, a reductive catalyst pretreatment before every test was chosen (5% H2 in He for 30 min at 150 oC) [15]. In respect to the analysis system and the appropriate calibration, the accuracy of the conversion and selectivity results was within 2 – 3% of the given value.
3. Results and discussion
3. 1. Catalytic measurements
The degree of CO conversion and selectivity in PROX over gold catalysts on alumina, ceria and mixed oxides supports prepared by IM or MM methods are illustrated in Figure 1. In all cases no trace of methane as a side product was registered. AuAl sample showed almost zero activity. Generally, the gold catalysts on CeO2/Al2O3 and Y-doped CeO2/Al2O3 supports prepared by MM exhibited better PROX behaviour as compared to the corresponding supports prepared by IM. These results are in agreement with the study of Carabineiro et al. [17] showing that gold catalysts on supports obtained by simple physical mixing of commercial alumina and different metal oxides (including CeO2) were much more active in CO oxidation than the gold catalysts on corresponding supports prepared by traditional way of impregnation. The positive effect of yttrium [15] was confirmed by the higher activity of AuY20CeAlIM then that of Au20CeAlIM catalyst. However, the yttrium-free Au20CeAlMM catalyst showed the highest CO conversion, equal to that of AuCe at 80 oC.
The activities of the catalysts at this temperature followed the row: AuCe=Au20CeAlMM>AuY20CeAlMM= AuY20CeAlIM>Au20CeAlIM. Catalytic test with 10% CO2 and 10% H2O addition to the gas feed was carried out over Au20CeAlMM, AuY20CeAlMM and AuY20CeAlIM samples (the Au20CeAlIM catalyst with the lowest CO conversion was not tested). The results are shown in Figure 2. The drop of PROX activity and selectivity at these conditions can be explained by the well-known detrimental effect, especially of CO2. It is related to the competitive adsorption between CO and CO2, the latest led to the carbonate and carboxylate species accumulation and blocking of the active sites [18,19].
The best performance in the presence of CO2 and water was obtained at 100 oC, following the line of sample activities: AuY20CeAlIM>Au20CeAlMM> AuY20CeAlMM. The long term test during 24 h at this temperature (Figure 3) showed more significant decrease with the time of CO conversion and selectivity over Au20CeAlMM catalyst, while Y-containing samples exhibited better stability.
3. 2. Catalyst characterization
The actual gold content (CAu), estimated by XRF analysis is given in Table 1. It is seen that the amount of gold on IM supports was closed to the nominal one, while on MM supports the CAu values were almost twice lower. These observations can be explained by the effect of supports preparation on the distribution of the CeO2 phase with respect to alumina: when applying IM method, the ceria layers covered alumina surface, while by MM ceria phase was randomly distributed and a large part of alumina surface remained free. The lower gold content on MM supports could then be related to the prevailing affinity of gold with ceria during the deposition precipitation procedure. The existence of ceria free alumina areas decreased the gold anchoring probability.
The values of specific surface area (SBET), mean pore diameter (dpore) and total pore volume (vpore) of gold catalysts on ceria/alumina and Y-doped ceria/alumina supports prepared by IM and MM are listed in Table 1. The textural characteristics of the supports prepared by MM method were similar to the used γ-alumina.
A larger decrease of SBET and vpore was observed in the case of the IM prepared supports.
The XRD patterns (Figure 4) of the studied catalysts showed crystal phases of CeO2 and γ-Al2O3 (not illustrated in a figure). The average size of ceria particles (Dceria) and the lattice parameter (aceria) are given in Table 1. The error was evaluated from the statistic regression error. For Dceria it was typically around 0.3 – 0.4 nm. For cell parameter aceria it is marked in parentheses.
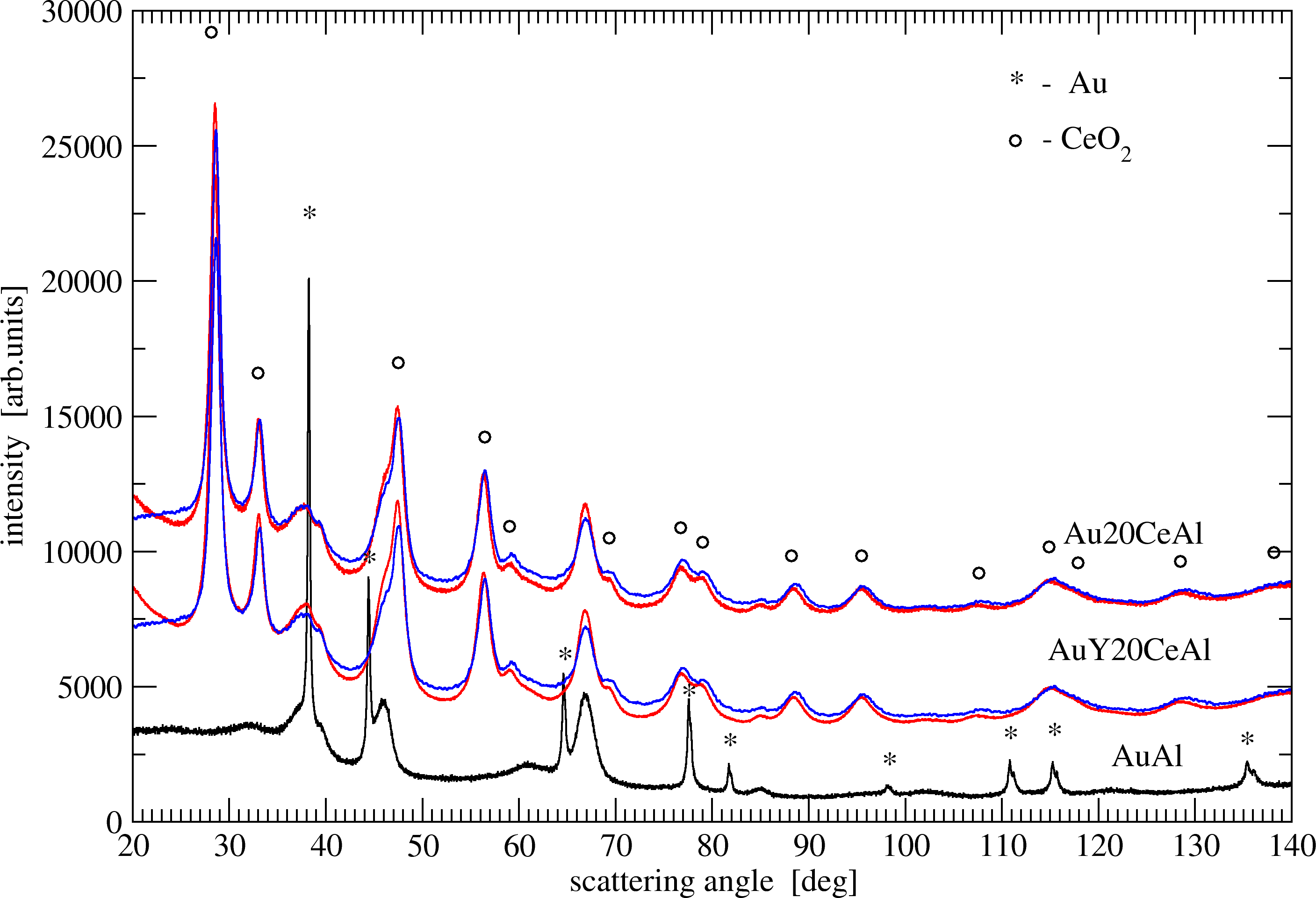
Table 1. Characteristic data of the studied samples (A): 1–γ-Al2O3, 2–Au20CeAlIM, 3–AuY20CeAlIM, 4–Au20CeMM and 5–AuY20CeMM
A | SBET m2/g |
vpore cm3/g |
dpore nm |
Dceria nm |
aceria Å |
CAu wt.% |
DAu nm |
1 | 200 | 0.53 | 10.6 | - | - | - | - |
2 | 149 | 0.36 | 9.6 | 11.4 | 5.398(1) | 2.86 | 1.9 |
3 | 150 | 0.37 | 9.3 | 11.4 | 5.399(1) | 2.88 | 1.6 |
4 | 172 | 0.45 | 10.3 | 6.6 | 5.409(2) | 1.64 | 2.2 |
5 | 172 | 0.43 | 10.1 | 6.2 | 5.410(2) | 1.66 | 3.1 |
Significantly smaller (almost half) average crystallite size of ceria was achieved by MM support preparation as compared to the case of impregnation. The values of ceria lattice parameter, obtained for the IM samples, were smaller than the lattice parameter of stoichiometric CeO2 (5.410 Å) [20,21] or Au/CeO2 (5.413 Å) [15]. The lattice contraction of the IM samples in comparison to the MM ones can be related to the difference in size. The increase of crystallites size was accompanied with a decrease in the lattice parameter as recently observed by Reina et al. [22]. The a values of undoped and Y-doped samples are quite close for both preparation methods. The reason is due to the proximity of the ionic radii (0.97 Å for Ce4+ and 1.02 Å for Y3+ in eight-fold coordination) whereat the replacement of Ce by Y ions does not contribute for substantial changing of lattice parameter.
The BET and XRD results (Table 1) revealed the influence of preparation method on the textural and structural features of ceria/alumina supports. The smaller ceria particles obtained using MM method entered the pores leading to slight lowering of surface area and pore volume as compared to alumina, while the mean pore diameter remained the same. Differently, ceria particles with average size bigger than the mean alumina pore diameter were obtained using impregnation. The large CeO2 particles blocked the pores resulting in a decrease of surface area, pore diameter and volume. The clogging pores hindered the access of reagents, causing a reduced reactivity of gold catalysts on IM as compared to MM ceria/alumina supports. These observations explained the better PROX behaviour of Au20CeAlMM and AuY20CeAlMM as compared to the corresponding IM catalysts.
Reflections of gold were registered only for AuAl sample and the calculated average size of gold particles was 44 nm. This observation was consistent with the extremely low activity in PROX. Reflections of gold and Y2O3 phase were not detectable by XRD for the other studied samples. The presence of separate Y2O3 crystals in the supports prepared by both methods were evidenced by HRTEM in the respective Fast Fourier transforms images with the interplanar distance of 2.499 Å that corresponds exactly to the distance reported in JCPDS data file 88-1040. Supplementary to the role of Y-modification of ceria, the presence of Au/Y2O3 could contribute for higher PROX activity according to the reported conclusion that Y2O3, stabilizing Au active species, improved the CO oxidation activity [23]. In this respect, the lower PROX activity of AuY20CeAlMM compared to that of Au20CeAlMM was not expected. The possible reason was searched in the dispersion of gold as a crucial factor for CO activation. The average size (Table 1) and the size distribution histograms (Figure 5) of gold particles were determined on the basis of HRTEM and Z contrast (HAADF) images. Small average size of gold particles between 2.0 and 2.4 nm was estimated in all cases except a higher value of 3.1 nm for AuY20CeMM catalyst. The size distribution histogram of this sample represented bigger fractions of particles larger than 2 nm and some particles larger than 10 nm were also registered (0.9 % of the measured particles). Differently, for Au20CeMM catalyst (average size of gold particles 2.2 nm) the percentage of particles from 1 to 1.99 nm was the highest (40%) and no particles larger than 10 nm were observed.
It is known that bigger Au particles adsorb relatively less CO [24]. Moreover, accepting the Mars–van Krevelen mechanism [7], the interface between gold and support is of crucial importance. The HRTEM analyses showed that apparently the gold particles deposited on Al2O3 crystals were larger than those deposited on CeO2. Such results in combination with the already commented lower gold loading on the supports prepared by MM could mean a reduced perimeter length between the gold particles responsible for CO activation and the supplying oxygen active ceria phase in the case of AuY20CeMM as compared to Au20CeMM catalyst. We supposed this as a reason for the unforeseen lower PROX activity over the Y-containing MM sample (Figure 1).
In the case of CO2 and water addition, the observed activity at 100 oC over Au20CeMM was slightly higher than that of AuY20CeMM but at higher temperatures the presence of yttrium showed a positive effect. The AuY20CeMM catalyst showed better performance above 100 oC than the Au20CeMM sample for which the highest CO conversion sharply decreased. The long term tests (Figure 3) revealed the role of Y-doping for better catalyst stability. It is reported in the literature that CeO2 has stronger basic properties as compared to Y2O3 [25]. The higher resistance to CO2 deactivation could be related to the Y2O3 (as commented above a separate Y2O3 phase was evidenced by HRTEM) covering ceria and lowering the surface basicity.
5. Conclusion
Gold catalysts on CeO2/Al2O3 and Y-doped CeO2/Al2O3 supports (20 wt.% of CeO2 and 1 wt.% Y2O3) prepared by mechanical mixing exhibited better PROX behaviour as compared to the corresponding samples deposited over supports obtained by impregnation (gas feed composition 60% H2 + 1% CO + 1% O2). The explanation was related to the effect of preparation method on the textural and structural properties of the supports. The MM method led to smaller ceria particles, which did not clog the pores of alumina. Differently, in case of IM technique the pore blocking by ceria particles with average size bigger than the mean pore diameter of alumina caused difficult access of reagents and therefore a reduced activity. The result of the highest CO conversion at 80 oC, equal to that of Au/ceria, over the yttrium-free MM catalyst was unexpected. The explanation was found in the key role of the interface between gold, responsible for CO activation, and ceria support supplying active oxygen. The results for actual gold loading and the HRTEM data revealed reduced length of the gold/ceria border for Y-doped MM catalyst. Adding CO2 and water to the gas stream, this sample exhibited again a slightly lower activity as compared to the undoped one at 100 oC but at higher temperatures the order of activities changed. The long-term test at realistic conditions in the presence of CO2 and water revealed the beneficial role of Y-doping for catalysts stability.
The results reported in the present study about gold catalysts deposited on CeO2/Al2O3 supports (with only 20 wt.% of CeO2) could contribute to the developing of active, selective and cost-efficient catalysts for hydrogen purification in PEMFC devices.
Acknowledgements
Authors affiliated at Institute of Catalysis, Bulgarian Academy of Sciences gratefully acknowledge financial support by the Bulgarian National Science Fund (Project DN 09/5/2016).
R. Zanella acknowledges the financial support granted by PAPIIT-UNAM (IN103719) and PDCPN-CONACYT (1216) and the technical support provided by V. Maturano.
Joint Italian–Bulgarian research project between CNR and BAS is also acknowledged.
References
[1] G. Bond, D. Thompson, “Catalysis by gold,” Cat. Rev-Sci. Eng., vol. 41, pp. 319-388, 1999. View Article
[2] M. Haruta, T. Kobayashi, H. Sano, N. Yamada,“Novel Gold Catalysts for the Oxidation of Carbon Monoxide at a Temperature far Below 0℃,“ Chem. Lett., vol. 16, p.p. 405-408, 1987. View Article
[3] M. Haruta, S. Tsubota, Kobayashi, H. Kageyama, M. Jenet, B. Delmon, “Low temperature CO oxidation over gold supported on TiO2, α-Fe2O3 and Co3O4,“J. Catal., vol. 144, pp. 175-192, 1993. View Article
[4] R. J. H. Grisel, C. J. Weststrate, A. Goossens, M. W. J. Craje, A. M. Van der Kraan, B. E. Nieuwenhuys, “Oxidation of CO over Au/MOx/Al2O3 multi-component catalysts in a hydrogen-rich environment,” Catal. Today, vol. 72, pp. 123-132, 2002. View Article
[5] M. Haruta, “Size-and support-dependency in the catalysis of gold,” Catal. Today, vol. 36, pp. 153-166, 1997. View Article
[6] W. Y. Hernández, M. A. Centeno, F. Romero-Sarria, J. A Odriozola, “Synthesis and characterization of Ce1−xEuXO2−x/2 mixed oxides and their catalytic activities for CO oxidation,” J. Phys. Chem. C, vol. 113, pp. 5629-5635, 2009. View Article
[7] L. F. Liotta, G. Di Carlo, G. Pantaleo, A. M. Venezia, “Supported gold catalysts for CO oxidation and preferential oxidation of CO in H2 stream: Support effect,” Catal. Today, vol. 158, pp. 56-62, 2010. View Article
[8] S. A. C. Carabineiro, S. S. T. Bastos, J. J. M. Órfão, M. F. R. Pereira, J. J. Delgado, J. L. Figueiredo, “Exotemplated ceria catalysts with gold for CO oxidation” Appl. Catal. A, vol. 381, pp. 50-160, 2010. View Article
[9] T. R. Reina, E. Papadopoulou, S. Palma, S. Ivanova, M. A. Centeno, T. Ioannides, J. A. Odriozola, “Could an efficient WGS catalyst be useful in the CO-PrOx reaction,” Appl. Catal. B, vol. 150–151, pp. 554-563, 2014. View Article
[10] T. R. Reina, S. Ivanova, M. I. Domínguez, M. A. Centeno, J. A. Odriozola, “Sub-ambient CO oxidation over Au/MOx/CeO2-Al2O3 (M = Zn or Fe),” Appl. Catal. A, vol. 419–420, pp. 58-66, 2012. View Article
[11 ] T. R. Reina, S. Ivanova, M. A. Centeno, J. A. Odriozola, “Low-temperature CO oxidation on multicomponent gold based catalysts,” Frontiers Chem., vol. 1; article 12, 2013. View Article
[12 ] T. R. Reina, S. Ivanova, M. A. Centeno, J. A. Odriozola, ”Catalytic screening of Au/CeO2-MOx/Al2O3 catalysts (M = La, Ni, Cu, Fe, Cr, Y) in the CO-PrOx reaction,” Int. J. Hydrogen Energy, vol. 40, pp. 1782-1788, 2015. View Article
[13] L. Ilieva, P. Petrova, G. Pantaleo, R. Zanella, J. W. Sobczak, W. Lisowski, Z. Kaszkur, G. Munteanu, I. Yordanova, L. F. Liotta, A. M. Venezia, T. Tabakova, “Alumina supported Au/Y-doped ceria catalysts for pure hydrogen production via PROX,” Int. J. Hydrogen Energy, vol. 44, pp. 233-245, 2019. View Article
[14] L. Ilieva, P. Petrova, G. Pantaleo, R. Zanella, J.W. Sobczak, W. Lisowski, I. Ivanov, Z. Kaszkur, L.F. Liotta, A.M. Venezia, T. Tabakova, “Impact of ceria loading on the preferential CO oxidation over gold catalysts on CeO2/Al2O3 and Y-doped CeO2/Al2O3 supports prepared by mechanical mixing,” Catal. Today, doi.org/10.1016/j.cattod.2019.06.006
[15] L. Ilieva, P. Petrova, G. Pantaleo, R. Zanella, L. F. Liotta, V. Georgiev, S. Boghosian, Z. Kaszkur, J. W. Sobczak, W. Lisowski, A. M. Venezia, T. Tabakova, “Gold catalysts supported on Y-modified ceria for CO-free hydrogen production via PROX,” Appl. Catal. B, vol. 188, pp. 154-168, 2016. View Article
[16] L. F. Liotta, G. Pantaleo, F. Puleo, A. M. Venezia, “Au/CeO2-SBA-15 catalysts for CO oxidation: Effect of ceria loading on physic-chemical properties and catalytic performances,” Catal. Today, vol. 187, pp. 10-19, 2012. View Article
[17] S. A. C. Carabineiro, P. B. Tavares, J. L. Figueiredo, “Gold on oxide-doped alumina supports as catalysts for CO oxidation,” Appl. Nanosci., vol. 2, pp. 35-46, 2012. View Article
[18] M. M. Schubert, V. Pizak, J. Garche, R. J. Behm, “Activity, selectivity and long-term stability of different metal oxide supported gold catalysts for the preferential CO oxidation in H2-rich gas,” Catal. Lett., vol. 76, pp. 143-150, 2001.
[19] A. Luengnaruemitchai, D. T. K. Thoa, S. Osuwan, E. Gulari, “A comparative study of Au/MnOx and Au/FeOx catalysts for the catalytic oxidation of CO in hydrogen rich stream,” Int. J. Hydrogen Energy, vol. 30, pp. 981-987, 2005. View Article
[20] M. Wolcyrz, L. Kepinski, “Rietveld refinement of the structure of CeOCl formed in Pd/CeO2 catalyst: Notes on the existence of a stabilized tetragonal phase of La2O3 in La-Pd-O system,” J. Solid State Chem., vol. 99, pp. 409-413, 1992. View Article
[21] S. R. Bishop, D. Marrocchelli, C. Chatzichristodoulou, N. H. Perry, M. B. Mogensen, H. L. Tuller, E. D. Wachsman, “Chemical expansion: implications for electrochemical energy storage and conversion devices,” Annu. Rev. Mater. Res., vol. 44, pp. 205-239, 2014. View Article
[22] T. R. Reina, S. Ivanova, V. Idakiev, J. J. Delgad., I. Ivanov, T. Tabakova, M. A. Centeno, J. A. Odriozola, “Impact of Ce–Fe synergism on the catalytic behavior of Au/CeO2-FeOx/Al2O3 for pure H2 production,” Catal. Sci. Technol., vol. 3, pp. 779-787, 2013. View Article
[23] J. Guzman, A. Corma, “Nanocrystalline and mesostructured Y2O3 as supports for gold catalysts,” Chem. Commun., pp. 743-745, 2005. View Article
[24] C. J. Weststrate, A. Resta, R. Westerstrom, E. Lundgren, A. Mikkelsen, J. N. Andersen, „CO Adsorption on a Au/CeO2 (111) Model Catalyst,“ Phys. Chem. C, vol. 112, pp. 6900-6906, 2008. View Article
[25] K. Tanabe, M. Misono, Y. Ono and H. Hattori, New Solid Acids and Bases: Their Catalytic Properties, Elsevier Science, 1989, pp. 18-23.