Volume 7 - Year 2019 - Pages 10-15
DOI: 10.11159/ijtan.2019.002
Fabrication and Surface-Modification of Silica/Gadolinium Compound/Silica Core-Shell Nanoparticles Discovered by Transmission Electron Microscopy
Eriko Kawamata, Tomoya Inose, Yoshio Kobayashi
Department of Materials Science and Engineering, Graduate School of Science and Engineering Ibaraki University
4-12-1 Naka-narusawa-cho, Hitachi, Ibaraki 316-8511, Japan yoshio.kobayashi.yk@vc.ibaraki.ac.jp
Abstract - The present work describes methods for fabricating multilayered core-shell nanoparticles composed of silica (SiO2) as a core, gadolinium compound (GdC) as an inner shell, and SiO2 as an outer shell (SiO2/GdC/SiO2) toward colloidally-stable, inert magnetic resonance imaging contrast agent, and for surface-modifying the SiO2/GdC/SiO2 nanoparticles toward immobilization of functional molecules on the SiO2/GdC/SiO2. SiO2 nanoparticles (particle size by transmission electron microscopy (TEM): 28.6±12.6 nm) were produced by a sol-gel method using tetraethylorthosilicate (TEOS) and methylamine in water/ethanol solution. GdC-coated SiO2 nanoparticles (SiO2/GdC) (GdC shell thickness by TEM: 35.9±4.9 nm) were synthesized by a homogeneous precipitation method using water/propanol solution containing the SiO2 nanoparticles, Gd(NO3)3, urea and polyvinylpyrrolidone. Succeeding SiO2-coating of the SiO2/GdC nanaoparticles was performed by using TEOS and NaOH in the presence of SiO2/GdC nanoparticles. Consequently, SiO2/GdC/SiO2 nanoparticles (SiO2 shell thickness by TEM: 8.5±2.7 nm) could be obtained. The SiO2/GdC/SiO2 nanoparticles were successfully surface-modified by using 3-aminopropyltrimethoxysilane for amination, and by followingly using succinic anhydride for carboxylation.
Keywords: Multilayer, Core-shell, Nanoparticle, Silica, Gadolinium, Sol-gel, Homogeneous precipitation, Surface-modification
© Copyright 2019 Authors - This is an Open Access article published under the Creative Commons Attribution License terms. Unrestricted use, distribution, and reproduction in any medium are permitted, provided the original work is properly cited.
Date Received: 2018-06-25
Date Accepted: 2018-09-17
Date Published: 2019-11-04
Introduction
Because of magnetic resonance based on paramagnetism of gadolinium (Gd) ions, they function to increase contrast of images taken by a magnetic resonance imaging (MRI) technique [1]. Commercially-available Gd ion-related MRI contrast agents are solutions dissolving the Gd complexes homogeneously at molecular level. The contrast agents injected into living bodies flow in living bodies, and finally are discharged out of the living bodies. Since Gd complex molecules are smaller than the particles, their velocity may be large compared to nano- or submicron particles, which provides small drag force given from fluid. Thus, the discharge for the Gd complex molecules is quick, which makes it difficult to take high contrast images for a long period.
Particles containing Gd compounds (GdC) are promising as a new MRI contrast agent. The particles, which are larger than Gd ion-related molecules, are dragged from fluid to a direction opposite to the fluid due to their projected area larger than molecules, so that they can be expected to stay in living bodies for a long period due to controlled flow of the particles in liquid. Consequently, formation of the particles containing GdC will increase residence time of GdC in the living bodies, which makes it possible to take clear MRI images for a long term. The particles are required to unaggregate in living bodies, because aggregation of the particles increases their apparent size, which prevents them from flowing in body fluid. Since surface of silica (SiO2) particles prepared by a sol-gel method has a feature of colloidal stability, SiO2 particles containing GdC may function as the MRI contrast agent that shows long residence time and non-aggregation in living bodies. Some researchers have studied on particles composed of GdC as core and silica as shell [2,3]. Our research group also has reported the fabrication of core-shell nanoparticles composed of core of GdC and shell of SiO2 (GdC/SiO2) [4].
Because strong interaction between water protons and GdC molecules promotes MRI properties of GdC [5], the GdC is desired to be present on or near surface of SiO2 nanoparticles containing GdC. Accordingly, core-shell particles containing GdC as core are not necessarily suitable to MRI contrast agents. Then, several researchers have studied on core-shell particles composed of SiO2 as core and GdC as shell [6,7], in which the colloidal stability of SiO2 particles will stabilize the core-shell particles colloidally. The GdC on or near the particle surface may release free gadolinium ions through dissociation of the complexes. The release of gadolinium ions may provoke adverse reactions in some patients [8], though their toxicity is lower than that of iodine compounds. As one of methods for reducing the adverse reactions derived from GdC, coating of GdC surface with materials inert to living bodies as shell can be given, because contact of the GdC with living bodies may be controlled with a physical barrier of the shell materials. The SiO2 is a candidate as the shell materials because of its inertness to living bodies. From this view point, our research group has reported the fabrications of core-shell nanoparticles composed of core of SiO2 and shell of GdC (SiO2/GdC), and of multilayered core-shell nanoparticles composed of core of SiO2/GdC nanoparticles and shell of SiO2 (SiO2/GdC/SiO2) [9]. The core-shell nanoparticles have another advantage. The GdC inside the particles can not function as the MRI contrast agent well, because it can not contact the protons in water. Thus, if the inside of the particles is replaced with core nanoparticles other than the GdC, the replacement will decrease the production cost of MRI contrast agent nanoparticles.
To use the MRI contrast agent nanoparticles practically, functional molecules such as polyethyleneglycol and antibody protein should be immobilized onto the particles for avoiding the particles from being recognized as foreign materials in living bodies and delivering the particles to antigen proteins due to a good affinity between the antibody proteins and the antigen proteins, respectively. Prior to the immobilizations, the particles are desired to be surface-modified with functional groups such as amino group and carboxylic group, because some functional molecules are attracted to these functional groups electro-statically or many kinds of chemicals react with the functional groups. The present paper describes methods for fabricating SiO2/GdC/SiO2 nanoparticles, and for surface-modifying SiO2/GdC/SiO2 nanoparticles toward a precursor of MRI contrast agent nanoparticles on which various functional molecules are immobilized.
2. Experimental
2. 1. Chemicals
Tetraethylorthosilicate (TEOS) (96%), aqueous methyl amine (MA) (40% solution) and ethanol (99.5%) were used for preparation of silica cores and silica-coating as monomer, catalyst and solvent, respectively. Gadolinium nitrate hexahydrate (Gd(NO3)3·6H2O) (99.5%), urea (99.0%), polyvinylpyrrolidone (PVP) (average molecular weight 36,000), and 1-propanol were used as chemicals for Gd shell, a precipitation-inducer, a stabilizer of particles for fabrication of SiO2/GdC nanoparticles, and solvent, respectively. A sodium hydroxide (NaOH) aqueous solution (1 M) was used as catalyst for formation of silica shells. (3-Aminopropyl)trimethoxysilane (APMS) (97%) and succinic anhydride (SA) (98.0%) were used for surface-modification of particles with amino groups and carboxyl groups, respectively. The solvent dissolving SA in the surface-modification with carboxyl groups was dimethylformamide (DMF) (99.5%). Salicylaldehyde (97.0%) was used for confirming the presence of amino groups in particle colloid solutions. The PVP and the APMS were purchased from Nacalai Tesque Ltd. (Kyoto, Japan) and Sigma-Aldrich Co. LLC (St. Louis, USA), respectively, all the other chemicals were purchased from Wako Pure Chemicals Ltd. (Osaka, Japan). All chemicals were used as received. Water that was ion-exchanged and distilled with Shimadzu SWAC-500 was used in all the preparations.
2. 2. Preparation of nanoparticles
A colloid solution of SiO2 nanoparticles was prepared as follows. A solution of TEOS and ethanol was added to a solution of MA, water and ethanol under stirring in a hermetically sealed flask with a magnetic stirrer. The reaction temperature and time were 35°C and 24 h, respectively. Initial concentrations of TEOS, H2O and MA were 0.2, 20, and 0.018 M, respectively. The silica nanoparticles were washed by repeating centrifugation, removal of supernatant, addition of the water and sonication over three times.
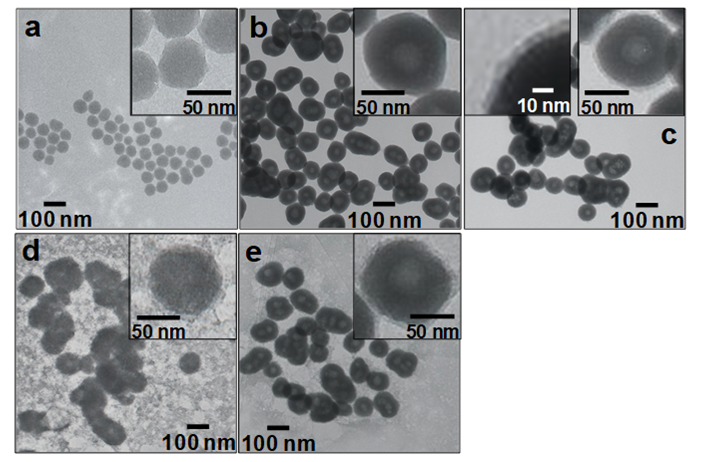
A colloid solution of SiO2/GdC nanoparticles was prepared as follows. The colloid solution of washed SiO2 nanoparticles and PVP dissolved in 1-propanol were added to a water/1-propanol solution, and the mixture was aged for 24 h at room temperature under stirring in a hermetically sealed flask with a magnetic stirrer. A urea aqueous solution was added to the aged mixture, and then pH of the mixture was adjusted to 5 by adding nitric acid. A Gd(NO3)3 aqueous solution was added to the mixture. The mixture was aged for 3 h at 80°C under stirring. Initial concentrations were 0.001 vol% SiO2, 1 g/L PVP, 0.5 M urea and 0.001 M Gd(NO3)3, and a volume ratio of water : 1-propanol was 1 : 1.
A colloid solution of SiO2/GdC/SiO2 nanoparticles was prepared as follows. The TEOS and the NaOH aqueous solution were added to the as-prepared colloid solution of SiO2/GdC nanoparticles under stirring in a hermetically sealed flask with a magnetic stirrer. The reaction temperature and time were 35°C and 24 h, respectively. Initial concentrations of TEOS and NaOH were 0.013 and 0.002 M, respectively.
2. 3. Surface-modification of nanoparticles
The SiO2/GdC/SiO2 nanoparticles were surface-modified with amino groups (SiO2/GdC/SiO2-NH2) as follows. The APMS was added to the as-prepared colloid solution of SiO2/GdC/SiO2 nanoparticles. The reaction temperature and time were 35°C and 24 h, respectively. An initial APMS concentration was 0.03 M. The SiO2/GdC/SiO2-NH2 nanoparticles were washed by repeating centrifugation, removal of supernatant, addition of solvent and sonication over two times. The solvents used in the second and the third washing processes were 1-propanol and DMF, respectively, which provided the replacement of solvent of colloid solution with DMF.
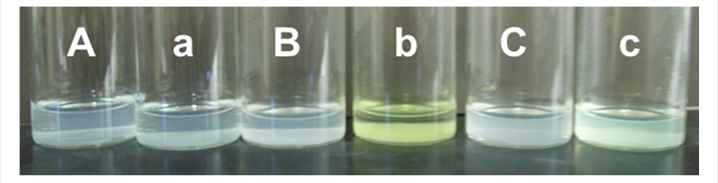
According to the reference [10], the amino groups on SiO2/GdC/SiO2-NH2 nanoparticles were transformed to carboxyl groups (SiO2/GdC/SiO2-COOH) as follows. The SA dissolved in DMF was added to the colloid solution of washed SiO2/GdC/SiO2-NH2 nanoparticles at 35°C. An initial SA concentration was 0.05 M. After 24 h, the SiO2/GdC/SiO2-COOH particles were washed by repeating centrifugation, removal of supernatant, addition of water and sonication over three times, which provided the replacement of solvent of colloid solution with water.
2. 4. Characterization
Morphology of the particles was investigated by transmission electron microscopy (TEM). TEM was performed with a JEOL JEM-2000FX II microscope operating at 200 kV. Samples for TEM were prepared by dropping and evaporating the nanoparticle suspensions on a collodion-coated copper grid. Dozens of particle diameters in TEM images were measured to determine volume-averaged particle size, dv, and standard deviation of particle size distribution, σ, defined by the following equations.


where ni is the number of particles with a size of di. The measurement of particle diameter was performed by using a Mitsutoyo CD-15AX digimatic caliper. The presence of amino groups in particle colloid solutions was confirmed by yellowish coloration provided by a reaction between salicylaldehyde and amino group [11].
3. Results and Discussion
3. 1. Morphology of nanoparticles
The colloid solution of SiO2 nanoparticles was prepared by a sol-gel method using TEOS as a SiO2 source and MA as a catalyst. Fig. 1 (a) shows a TEM image of the SiO2 nanoparticles. The silica nanoparticles were quasi spherical and were colloidally stable. They had an average size of 28.6±12.6 nm by TEM. The SiO2/GdC nanoparticles exhibited no aggregation during the GdC-coating of SiO2 nanoparticles, which indicated that colloidal stability of SiO2 nanoparticles prevented generation of aggregation.
The GdC-coating of SiO2 nanoparticles, or fabrication of SiO2/GdC nanoparticles was performed by a homogeneous precipitation method in the presence of the washed silica nanoparticles. Fig. 1 (b) shows a TEM image of SiO2/GdC nanoparticles. The SiO2 nanoparticles were coated with uniform GdC shell. An average particle size and an average shell thickness were 107.0±23.0 and 35.9±4.9 nm, respectively, by TEM. There was neither uncoated SiO2 nanoparticles nor core-free particles.
The SiO2-coating of SiO2/GdC nanoparticles, or fabrication of SiO2/GdC/SiO2 nanoparticles was performed by a sol-gel method using TEOS as a SiO2 source and NaOH as a catalyst. Fig. 1 (c) shows a TEM image of SiO2/GdC/SiO2 nanoparticles. Prior to the TEM observation, the nanoparticles were washed by repeating centrifugation, removal of supernatant, addition of the water and sonication over three times. Only multilayered core-shell particles were observed, and there was neither SiO2/GdC core-free silica nor SiO2 shell-free GdC/SiO2 nanoparticles. An average particle size was 119.0±39.0 nm by TEM. An average SiO2 shell thickness was 8.5±2.7 nm, according to a high magnification image (a left inset of Fig. 1 (c)). It was confirmed by naked eye that no sedimentation took place for the SiO2/GdC/SiO2 nanoparticle colloid solution. This observation indicated that the SiO2 shell contributed to their colloidal stability.
3. 2. Surface-modification
The SiO2/GdC/SiO2 nanoparticles were surface-modified with amino groups by a reaction between an alkoxide group of APMS and hydroxide groups of the SiO2 surface on SiO2/GdC/SiO2 nanoparticles. Fig. 1 (d) shows a TEM image of SiO2/GdC/SiO2-NH2 nanoparticles. Their multilayered core-shell structure of SiO2/GdC/SiO2 nanoparticles was not damaged even with the surface-modification process using APMS. Many gel networks were also generated. Since the as-prepared SiO2/GdC/SiO2 nanoparticles were used for the surface-modification, unreacted TEOS that did not form the SiO2 shells had might been left in the colloid solution. Probably, SiO2 nuclei were generated through hydrolysis and condensation of alkoxide groups of TEOS. Generation of silanol groups through the hydrolysis probably increased the ionic strength. Increased ionic strength has been reported to compress the double layer of colloidal particles [12]. The generation of silanol groups therefore decreased the double-layer repulsion between the nuclei. The increased ionic strength was thus primarily responsible for the aggregation and growth of nuclei in the present system, which formed the gel networks. A reaction between salicylaldehyde and amino group provides yellowish coloration of solution [11]. In the present work, the presence of amino groups in solutions was confirmed by the yellowish coloration. Figs. 2 (B) and (b) shows photographs of the SiO2/GdC/SiO2-NH2 nanoparticle colloid solutions prior to and after the addition of salicylaldehyde. The color of the colloid solution turned yellowish after the addition. In the case of the SiO2/GdC/SiO2 nanoparticles, no color change took place, as shown in Figs. 2 (A) and (a). Probably, the color change took place by a reaction between salicylaldehyde and amino group. Accordingly, the color change confirmed that amino groups were introduced on the SiO2/GdC/SiO2 particle surface, or the SiO2/GdC/SiO2 particle surface was successfully surface-modified with amino groups with the amination process.
The amino groups on SiO2/GdC/SiO2-NH2 nanoparticles were transformed to carboxyl groups by a reaction between a functional group of SA and the amino group on SiO2/GdC/SiO2-NH2 nanoparticles, so that the particles were expected to be surface-modified with carboxyl groups. Fig. 1 (e) shows a TEM image of SiO2/GdC/SiO2-COOH nanoparticles. Their multilayered core-shell structure of SiO2/GdC/SiO2-NH2 nanoparticles was not damaged even with the surface-modification process using SA. The amount of the gel network remarkably diminished compared to the SiO2/GdC/SiO2-NH2. Its weight was so light compared with the SiO2/GdC/SiO2-COOH nanoparticles that some of the gel networks did not sediment with the centrifugation process. Consequently, the repeated washing process gradually removed the non-sedimenting gel networks. Figs. 2 (C) and (c) shows photographs of the SiO2/GdC/SiO2-COOH nanoparticle colloid solutions prior to and after the addition of salicylaldehyde. The color of the colloid solution turned yellowish after the addition. The color change was slighter than that for SiO2/GdC/SiO2-NH2 nanoparticles. The amino groups were transformed to carboxyl groups by a reaction between the functional groups of SA and the amino groups on SiO2/GdC/SiO2-NH2 nanoparticles, which reduced the amino groups on particles. The reduction provided the slight color change, which indicated successful introduction of carboxyl groups onto particles, or surface-modification of particles with carboxyl groups.
4. Conclusion
Fabrication of multilayered SiO2/GdC/SiO2 core-shell nanoparticles and surface-modification of the multilayered core-shell nanoparticles have been performed toward colloidally-stable, inert magnetic resonance imaging contrast agent. The SiO2 nanoparticles with the size of 28.6±12.6 nm (by TEM) fabricated through the sol-gel method were coated with GdC shells by means of the homogeneous precipitation method with the aid of stabilizer PVP, which produced the SiO2/GdC nanoparticles that had the average GdC shell thickness of 35.9±4.9 nm (by TEM). The SiO2/GdC nanoparticles were coated with SiO2 shells by the sol-gel method using the as-prepared SiO2/GdC nanoparticle colloid solution, which formed the SiO2/GdC/SiO2 nanoparticles that had the average SiO2 shell thickness of 8.5±2.7 nm (by TEM). The SiO2/GdC/SiO2 nanoparticle surface was aminated with APMS, and the amino groups of nanoparticle surfaces were transformed to carboxyl groups with SA, which aimed immobilization of functional molecules on the SiO2/GdC/SiO2 core-shell nanoparticles.
References
[1] L. Telgmann, M. Sperling and U. Karst, “Determination of gadolinium-based MRI contrast agents in biological and environmental samples: A review,” Anal. Chim. Acta, vol. 764, pp. 1-16, 2013. View Article
[2] K. Kubasiewicz, M. Runowski, S. Lis and Agata Szczeszak, “Synthesis, structural and spectroscopic studies on GdBO3:Yb3+/Tb3+@SiO2 core-shell nanostructures,” J. Rare Earth, vol. 33, pp. 1148-1154, 2015. View Article
[3] S. Kumar, V. K. Meena, P. P. Hazarib and R. K. Sharma, “FITC-Dextran entrapped and silica coated gadolinium oxide nanoparticles for synchronous optical and magnetic resonance imaging applications”, Int. J. Pharmaceut., vol. 506, pp. 242-252, 2016. View Article
[4] H. Morimoto, M. Minato, T. Nakagawa, M. Sato, Y. Kobayashi, K. Gonda, M. Takeda, N. Ohuchi and N. Suzuki, “X-ray imaging of newly-developed gadolinium compound/silica core-shell particles”, J. Sol-Gel Sci. Technol., vol. 59, pp. 650-657, 2011. View Article
[5] R. Artali, G. Bombieri, G. B. Giovenzana, M. Galli, L. Lattuada and F. Meneghetti, “Preparation, crystallographic and theoretical study on a bifunctional Gd-AAZTA derivative as potential MRI contrast agent precursor,” Inorg. Chim. Acta, vol. 407, pp. 306-312, 2013. View Article
[6] V. Feldmann, J. Engelmann, S. Gottschalk and H. A. Mayer, “Synthesis, characterization and examination of Gd[DO3A-hexylamine]-functionalized silica nanoparticles as contrast agent for MRI-applications”, J. Colloid Interface Sci., vol. 366, pp. 70-79, 2012. View Article
[7] F. Liu, X. He, L. Liu, H. You, H. Zhang and Z. Wang, “Conjugation of NaGdF4 upconverting nanoparticles on silica nanospheres as contrast agents for multi-modality imaging”, Biomater., vol. 34, pp. 5218-5225, 2013. View Article
[8] G. Marshall and C. Kasap, “Adverse events caused by MRI contrast agents: implications for radiographers who inject”, Radiography, vol. 18, pp. 132-136, 2012. View Article
[9] Y. Kobayashi, J. Imai, D. Nagao, M. Takeda, N. Ohuchi, A. Kasuya and M. Konno, “Preparation of multilayered silica-Gd-silica core-shell particles and their magnetic resonance images,” Colloids Sur. A, vol. 308, pp. 14-19, 2007. View Article
[10] Y. An, M. Chen, Q. Xue and W. Liu, “Preparation and self-assembly of carboxylic acid-functionalized silica,” J. Colloid Interface Sci., vol. 311, 507-513, 2007. View Article
[11] F. Cuoq, A. Masion, J. Labille, J. Rose, F. Ziarelli, B. Prelot, J.-Y. Bottero, “Preparation of amino-functionalized silica in aqueous conditions,” Appl, Surf, Sci., vol. 266, pp. 155-160 (2013). View Article
[12] K. Dimic-Misic, M. Hummel, J. Paltakari, H. Sixta, T. Maloney and P. Gane, “From colloidal spheres to nanofibrils: extensional flow properties of mineral pigment and mixtures with micro and nanofibrils under progressive double layer suppression,” J. Colloid Interface Sci., vol. 446, pp. 31-43, 2015. View Article