Volume 4 - Year 2016 - Pages 1-8
DOI: 10.11159/ijtan.2016.001
Synthesis and Characterization of Polyaniline/Magnetite Nanocomposite
Rurik Farias-Mancilla1, José T. Elizalde-Galindo1, Enrique Vigueras-Santiago2, Claudia A. Hernández-Escobar3, Alejandro Vega-Rios3, E. Armando Zaragoza-Contreras3
1Universidad
Autónoma de Ciudad Juárez.
Av. del Charro 450 Norte. Cd. Juárez, Chihuahua, México
rurik.farias@uacj.mx; jose.elizalde@uacj.mx
2Universidad
Autónoma del Estado de México
Paseo Colón
Esquina Paseo Tollocan s/n, CP 50000 Toluca, Estado de México, México
enriquevigueras@yahoo.com.mx
3Centro de
Investigación en Materiales Avanzados, S.C.,
Miguel de
Cervantes No. 120, Complejo Industrial Chihuahua, Chihuahua, Chih., México
claudia.hernandez@cimav.edu.mx;
alejandro.vega@cimav.edu.mx; armando.zaragoza@cimav.edu.mx
Abstract - A conducting-electroactive polyaniline/magnetite (PAni/Fe304) nanocomposite was synthesized using anilinium dodecylbenzene sulfonate (S1) as a reactive surfactant. First, S1 allowed magnetite dispersion in the aqueous phase and second, S1 performed as the monomer of polyaniline emeraldine base salt. Electron microscopy suggested core-shell morphology based on S1 amphiphilic character; that is, S1 adsorbed onto the magnetite nanoparticles surface and then was polymerized via an oxidative polymerization forming the shell. The PAni/Fe304 composite exhibited improved thermal stability regarding pure PAni, which was related to the strong interaction between PAni and magnetite. Electrical conductivity, determined by the four-probe method, was in the order of 10-1 and 10-3 S cm-1, respectively, for the pure PAni and the composite. Concerning composite magnetic properties, the decrement in magnetization (σr) and hysteresis (Hc) was attributed to the increment in dipolar magnetic interaction due to the increased separation among magnetite nanoparticles because of the PAni shell.
Keywords: Conducting polymer, Nanocomposite, Magnetite, Polyaniline, Reactive surfactant.
© Copyright 2016 Authors - This is an Open Access article published under the Creative Commons Attribution License terms. Unrestricted use, distribution, and reproduction in any medium are permitted, provided the original work is properly cited.
Date Received: 2014-11-25
Date Accepted: 2015-06-05
Date Published: 2016-01-21
1. Introduction
Magnetic polymer nanocomposites represent a class of functional materials, where magnetic nanoparticles are embedded in polymer matrices [1]-[3]. These nanocomposites hold great potential for applications in, for example, storage information, power distribution, microwave communication, permeability and microwave absorption, electromagnetic device application, shape-memory, and electromagnetic interference suppression [4]-[7]. Conducting polymers have received special attention because of their excellent electrical properties and tunable conductivities [8]. Particularly, polyaniline (PAni) has been subject of intense study during the past decades because of its excellent electrical and chemical properties [9]. PAni displays novel properties such as special doping mechanisms and high environmental stability [10]. Furthermore, metal oxides with the spinel structure constitute an important class of materials exhibiting a wide variety of electrical, magnetic, and optical properties [11]. Magnetite (Fe3O4) is a common ferrite-possessing material with a cubic inverse spinel structure. Stable magnetite with high crystallinity and magnetic responses can be easily manufactured [12].
The study of PAni composites containing magnetite has been extensive; these materials are usually investigated due to their unique magnetic and electrical characteristics; for example, Xiao et al. [13] synthesized PAni/nano-Fe3O4 composites prepared in a Fe3O4 nanoparticle stabilized Pickering emulsion. They found that composite morphology and room-temperature conductivity depended not only on the volume ratio of toluene to water, but also on Fe3O4 loading in the reaction system. Chung et al. [14] reported the fabrication of PAni hollow spheres using polystyrene particles as the template. Subsequently, they synthesized PAni/Fe3O4 nanocomposites with Fe3O4 on the surface of the PAni hollow cluster structures by in-situ and ex-situ methods. The hollow morphology of the PAni particles, after removing polystyrene with acetone, was characterized by electron microscopy. The Fe3O4 nanoparticles synthesized with the ex-situ method were more widespread on the surface of PAni hollow spheres as compared with their counterparts synthesized with the in-situ method. De Araújo et al. produced polyaniline-Fe3O4 nanocomposite using Fe3O4 nanoparticles as the oxidizing agent. Conductivity for the pure magnetite and the nanocomposites were, respectively, in the order of 10-6 and 10-5 S cm-1, confirming conductivity increment as a function of PAni loading. Furthermore, the magnetic measurements showed ferromagnetic behavior for the nanoparticles, with high-saturated magnetization (MS = 74.30emu g−1) and a coercive force of 93.40 Oe [15]. Jacobo et al. reported a process for the preparation of composite films consisting of Fe3O4 nanoparticles embedded in a PAni matrix. The films showed both reasonably high electrical conductivity and magnetic permeability. Application of an external magnetic field during composite synthesis stimulated creation of magnetic particle aggregates which, although kept conductivity at a relatively high level left to a small decrease of the conductivity value [16]. Aphesteguy et al. reported the preparation of a processible Fe3O4/PAni nanocomposite, using dodecylbenzen sulfonic acid (DBSA) as a surfactant and dopant. The nanocomposite showed at 300 K no loop of hysteresis indicating the superparamagnetic nature. Whereas the electrical conductivity of the composites was higher than that of the pure PAni despite of the insulating character of the magnetite particles inserted [17]. Basavaiah et al. prepared magnetic polyaniline/magnetite nanocomposites by an in situ self-assembly method in the presence of DBSA as dopant and surfactant. The nanocomposite morphology was dependent on the molar ratio of DBSA to aniline [18]. Additionally, the nanocomposites showed superparamagnetism and high thermal stability. Ding et al. produced nanocomposites consisting of PAni nanorods surrounded by Fe3O4 nanoparticles. They used DBSA as the dopant and as surfactant in the reaction system. DBSA allowed the control of the morphology and size of PAni nanorods and magnetite particles. Magnetite particles were formed simultaneously during sedimentation, and the formed nanorods were also decorated by the particles [19]. Radhakrishnan et al. reported the synthesis and characterization of DBSA doped polyaniline (PAni) and its magnetite composites. The composites showed excellent dispersibility in water and organic solvents. The composites were evaluated as electrode materials for supercapacitor applications; they found that the single electrode of composite and the symmetrical capacitor based on it showed, respectively, enhanced capacitance of 228 and 180 F g-1 at 1 mA cm-1. Besides, the capacitance values increased with decreased magnetite loadings [20]. Khorshidi et al. prepared magnetic and conducting polyaniline/Fe3O4 nanoparticles in aqueous solution by using the sodium salt of DBSA as the surfactant. Composites conductivity decreased as a function of Fe3O4 content, producing values of 2.7 * 10-5 and 3.4 * 10-6 S cm-1, respectively, for loadings of 1 and 5 g L-1 of magnetite. Composite magnetic properties showed superparamagnetic behavior, such as saturated magnetization (Ms = 1.8 emu g-1), remnant magnetization (Mr = 0), and coercive force (Hc = 0) [21]. Recently, application of polyaniline/Fe3O4 composites in liquefied petroleum gas sensing [22], heavy-metal ion absorption [23], or microwave absorption [24] demonstrate the scientific interest for these materials and stimulates the exploration of new strategies of synthesis and new applications for future technologies.
As noted in the literature, there are a variety of strategies reported to obtain polyaniline/magnetite composites; the use of dodecylbenzene sulfonate as the doping agent and to control composite morphology and to provide solvent dispersibility is one. Taking inspiration from this concept, herein we report on the use of anilinium dodecylbenzene sulfate (S1) as a reactive surfactant; that is, S1 performs as a surfactant and is the monomer of polyaniline concurrently, Figure 1. As a surfactant, S1 assists in the dispersion of the magnetite nanoparticles in the aqueous phase and helps to counteract nanoparticles tendency to reagglomeration. As a monomer, through an oxidative polymerization, S1 produces conducting polyaniline (emeraldine base salt). Consequently, by using S1 both magnetite dispersion and polyaniline shell synthesis is possible, producing a core-shell composite with magnetic, electro-conductive, and electro-active properties.
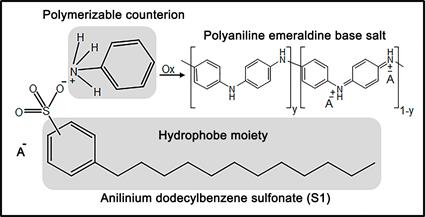
2. Experimental Section
2. 1. Synthesis of Fe3O4 Nanoparticles
Magnetite (Fe3O4) was synthesized following the method reported by Vergés et al. [12], this method used the FeSO4 salt precipitation in the presence of a base (NaOH) and a mild oxidant (KNO3). Optimal conditions for this synthesis were at concentrations of: a) ferrous solution containing 0.1 M FeSO4·7H20 and 0.01 M H2SO4; b) oxidation solution containing 0.07 M NaOH and 0.1 M KNO3. The two solutions were mixed by adding ferrous solution to the first solution at a constant rate of 60 mL min-1, under stirring and inert atmosphere (N2) reaching pH >11 during the reaction; a green phase of iron salt was obtained in the solution. After, the solution was thermally aged at 90 °C during 24 h to obtain a magnetite stable phase. Then, the solution was cooled down at room temperature; the precipitate obtained was magnetically decanted, washed, and dried.
2. 2. Synthesis of Polyaniline/Fe3O4 Composites
Magnetite/polyaniline composites were synthesized as follows: First, an aqueous dispersion of magnetite, in the presence of S1, was prepared using a dismembrator (Model 505, Fisher Scientific) programmed to apply pulses at 100 % amplitude every 2 s for 60 min. Afterwards, an aqueous solution of ammonium persulfate (APS) at a molar ratio APS to S1 of 1.2:1.0 was added dropwise over a period of 30 min. The oxidative polymerization was left at -2 °C for 24 h.
2. 3. Characterization
Scanning electron microscopy in transmission mode (STEM) was performed in a field emission electron microscope (JSM−7401F; JEOL) at 30 KV. To prepare the samples, a drop of aqueous dispersion, of magnetite or magnetite/polyaniline composite, was dispersed by sonication for 5 min in 30 mL of distilled water. Subsequently, a drop of dispersion was placed and left dry on a holey-carbon-cooper grid. X ray diffraction (XRD) pattern of the pure magnetite was obtained at room temperature using an X-ray diffractometer (Expert Pro, PANAlytical) with Cu-α radiation. All peaks were identified with the magnetite cubic crystalline phase (PDF-3 01-075-0449). The composite was characterized using a thermogravimetric analyzer (TGA Q500, TA Instruments). Measurement was achieved using 10 mg of sample and heated from laboratory temperature to 950 °C, at a heating rate of 10 °C min-1 under air atmosphere. Composite conductivity was determined by the four-probe technique. Assays were achieved on probes of 10 mm in diameter * 0.2 mm wide, prepared by compression at laboratory conditions. The PAni/Fe3O4 composite was characterized by cyclic voltammetry (CV) using a potentiostate analyzer (model 1260 plus 1287, Solartron). Electrochemical measurements were performed in a standard three-electrode cell at room temperature, using Pt square foil (area=0.75 cm2) as the counter electrode, and Ag/AgCl/saturated KCl as the reference electrode. The electrolyte was a sulfuric acid (H2SO4) solution 0.1 M. The analysis was performed at a scan rate of 50 mV s−1 by sweeping the potential from − 500 to +1000 mV against Ag/AgCl reference electrode. Working electrode was made with carbon paste depositing 5 mg of composite. Magnetic measurements were run at room temperature using a magnetometer (Versalab Crio Free VSM, Quantum Design) with maximum applied field Hmax= 20 kOe.
3. Results and Discussion
3. 1. Morphology
Figure 2(a) portrays an image of electron microscopy of the pure magnetite nanoparticles. As observed, the sample consists of nanometric semi-spherical particles with wide polydispersity. Concerning the composites, Figure 2(b and c), the presence of the polyaniline is observed as the grey cloud embedding the magnetite nanoparticles. In these, the intimate interaction between both materials is evident. This result is explained in terms of the surface activity of S1; that is, as S1 is a surfactant it is adsorbed onto the magnetite nanoparticles creating an interphase that reduces nanoparticle reagglomeration. When the oxidizing agent is added, S1 polymerization is achieved forming a layer of polyaniline on the nanoparticle surface. Consequently, S1 character both as a surfactant and monomer of polyaniline was evidenced, and the core-shell microstructure of the composite was assumed. Figure 2d shows magnetite nanoparticles statistical data. Average particle size (DAV), considering nanoparticles as spheres, is 17.7 nm. According to the analysis and considering a standard deviation (σ) of 7.5 nm, 72% of the nanoparticles are close to the most probable particle size, in this case 12.5 nm.
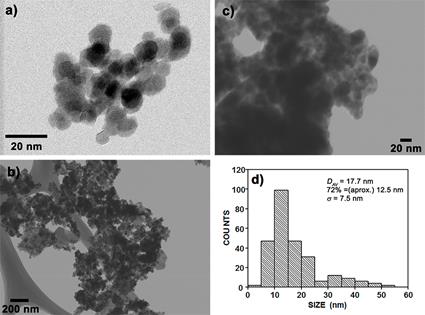
3. 2. Magnetite Crystalline Structure
Figure 3 shows the XRD pattern of the pure magnetite. The diffraction peaks at 2q = 30.2, 35.6, 43.3, 53.5, 57.2, and 63.03 correspond to (220), (311), (400), (422), (511), and (440) crystallographic planes of the spinel phase of Fe3O4, respectively; this pattern is in accordance with literature reported [25]. As noted, no peaks of any secondary phase were observed. Average crystallite size was calculated using Scherrer Equation (1):
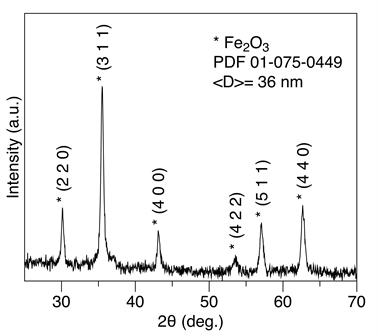
where λ is the X-ray wavelength (λ = 0.154 nm), <D> is the average crystallite size, κ is the dimensionless shape factor, β is the full-width at half the maximum (FWHM), and ⊖ is the Bragg angle in degree. The shape factor κ is normally 0.89. Using the FWHM of the most intense peak (2⊖ = 35.6) giving <D> = 36 +/‒ 1 nm. UNITCELL software was used to calculate the lattice parameters. Values of a = 8.370(1) Å resulted, which indicates that lattice parameter is not affected by synthesis process [26].
3. 3. Thermal Stability
Figure 4 shows thermogravimetric analysis traces of the pure polyaniline and the PAni/Fe3O4 composite. Concerning polyaniline, the initial weight loss above 150 °C is ascribed to water evaporation and to degradation of low molecular weight species (for instance, unattached dopant or PAni oligomers). The second mass loss close to 250 °C was associated with the doping agent degradation. Subsequently, the transition at 350 °C was attributed to polyaniline backbone decomposition [27], [28]. Concerning the composite, the first transition is equivalent to that observed in the pure PAni; however, the second and third transitions appeared, respectively, at 325 °C and 470 °C; that is, 75 °C and 100 °C higher than in the pure PAni. Such enhanced thermal stability was related to the strong interfacial interaction between PAni and magnetite, which restricts thermal motion of PAni chains [18], [29], [30]. Finally, it is worth mentioning, that the analysis indicated a composite composition close to 50 wt% of PAni and magnetite. This value indicates a high conversion of S1 to PAni, as in the initial formulation a weight ratio of 1:1 (wt/wt) of S1 to magnetite was used.
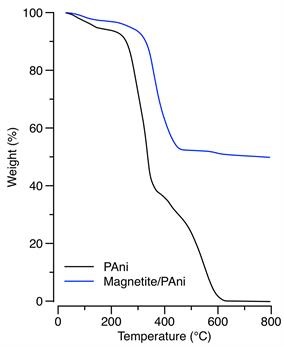
3. 4. Electrical Properties
Electro-conductivity of the pure PAni and the PAni/Fe3O4 composite was, respectively, 3.08 x 10-1 and 3.51 x 10-3 S cm-1. Concerning the pure magnetite, we had no result as an adequate tablet to achieve this measurement was not obtained. Considering literature, the usual behavior is the reduction of electro-conductivity at high magnetite loadings, such behavior has been related to the charge transport mechanism [31], [32]. Similar behavior has been reported for other magnetic materials [33], [34].
Figure 5 shows voltammograms of the pure polyaniline, magnetite, and PAni/Fe3O4 composite. As expected, magnetite showed no electrochemical response. Concerning the pure PAni and the composite, during cycling the positive range was swept from the half-doped state, called emeraldine, to fully the doped state, called pernigraniline. Furthermore, the negative range was swept from half-doped state to fully dedoped state, called leucoemeraldine. Both leucoemaraldine and pernigraniline states are non-conducting in nature, and offer high resistance. At high cell voltage, PAni does not have sufficient ion exchange sites to get doped; hence, a decrease in the current above 0.6 V occurred. According to literature, polyaniline voltammetric responses to acidic media has two coupling redox potentials for the range of -0.2 V < V < 1 V [35]. In the potential range from -0.2 to 1 V, the compounds show a redox couple manifested as two voltammetric peaks. These peaks are not symmetric regarding the shape or position on the potentials axis. The cathodic peak is always wider and appears at inferior potential values.
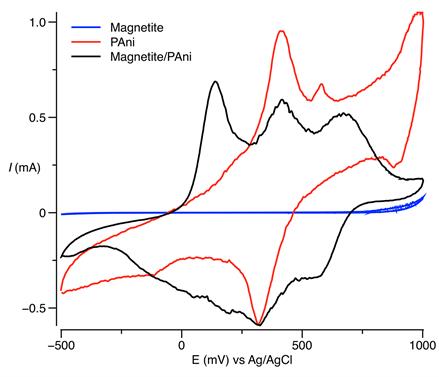
3. 5. Magnetic Properties
Figure 6 shows the hysteresis loop measured at room temperature with a Hmax of 20 kOe for Fe3O4 and PAni/Fe3O4 composite. The saturation magnetization value (σs) for Fe3O4 and PAni/Fe3O4 composite were, respectively, 58 and 50 emu g-1. These values are low contrasting with the reported theoretical saturation magnetization in magnetite (92 emu g-1), and to the value of commercial magnetite fine powder (84.5 emu g-1) [36]. For the Fe3O4 nanoparticles, the lowering on the saturation magnetization could be attributable to morphology and superficial effects such as oxidation differences. Another reason could be a lack of symmetry at the surface, which yields to broken ligands. This caused a deficiency in super-exchange interaction between close-to-surface iron atoms. In addition, if a superficial oxygen layer is formed, the linked electrons cannot participate in super-exchange anymore, resulting in a decrease of net magnetization [37].
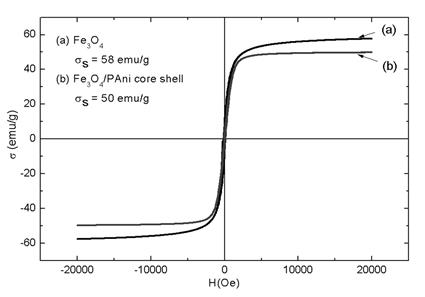
Meanwhile, for the PAni/Fe3O4 composite, the reduction in σs was attributed to the reduction in magnetic mass, as PAni is not contributing to magnetization values. Since the critical diameter for superparamagnetic behavior in Fe3O4 is 20 nm, and because the average crystallite size reached for our Fe3O4 nanoparticles is 36 nm, a ferrimagnetic behavior in both samples was expected [11]. Consequently, the remnant magnetization between 8 and 4 emu g-1 and coercivity between 100 and 60 Oe were exposed. The lower values of σr and Hc observed in PAni/Fe3O4 composite were attributed to the increment in dipolar magnetic interaction due to PAni shell formed onto the Fe3O4 nanoparticles surface.
4. Conclusion
S1 was successfully used for the synthesis of PAni/Fe3O4 composites. First, S1 facilitated the dispersion of the Fe3O4 nanoparticles in the reaction medium and then, through an oxidative polymerization, produced the shell providing the composite with conductive-electroactive properties. The PAni/Fe3O4 composite exhibited enhanced thermal stability compared to the pure PAni, which evidence the strong interfacial interaction between both components. Concerning electrical conductivity, values of 10-1 and 10-3 S cm-1 for the pure PAni and the PAni/Fe3O4 composite, respectively, obeyed the typical behavior reported for similar systems. The Fe3O4 nanoparticles exposed a ferrimagnetic behavior, with a saturation magnetization of 58 emu g-1. After formation of the PAni shell, the magnetic properties shifted to lower values due to magnetic mass reduction and to the enhanced magnetic dipolar interactions because of the separation between Fe3O4 nanoparticles.
Acknowledgement
We gratefully acknowledge financial support by PROMEP-SEP, Program: Redes Temáticas de Colaboración Académica, for "Red de Compuestos Poliméricos, Propiedades y Aplicaciones". We also wish to thank to Laboratorio Nacional de Nanotechnología (CIMAV), and to Wilber Antunez and Carlos Ornelas for their helpful collaboration during this research.
References
[1] X. Liu, Y. Guan, Z. Ma, and H. Liu, "Surface modification and characterization of magnetic polymer nanospheres prepared by miniemulsion polymerization," Langmuir, vol. 20, no. 23, pp. 10278-82, 2004. View Article
[2] I. Csetneki, M. K. Faix, A. Szilágyi, A. L. Kovács, Z. Németh, and M. Zrinyi, "Preparation of magnetic polystyrene latex via the miniemulsion polymerization technique," J. Polym. Sci. Part A Polym. Chem., vol. 42, no. 19, pp. 4802-4808, 2004. View Article
[3] G. Qiu, Q. Wang, C. Wang, W. Lau, and Y. Guo, "Polystyrene/Fe3O4 magnetic emulsion and nanocomposite prepared by ultrasonically initiated miniemulsion polymerization," Ultrason. Sonochem., vol. 14, pp. 55-61, 2007. View Article
[4] R. Mohr, K. Kratz, T. Weigel, M. Lucka-Gabor, M. Moneke, and A. Lendlein, "Initiation of shape-memory effect by inductive heating of magnetic nanoparticles," PNAS, vol. 103, pp. 3540-3545, 2006. View Article
[5] L. A. Dobrzański, M. Drak, and B. Ziębowicz, "New possibilities of composite materials application—Materials of specific magnetic properties," J. Mater. Process. Technol., vol. 191, pp. 352-355, 2007. View Article
[6] I. Kong, S. H. Ahmad, M. H. Abdullah, D. Hui, A. N. Yusoff, and D. Puryanti, "Magnetic and microwave absorbing properties of magnetite-thermoplastic natural rubber nanocomposites," J. Magn. Magn. Mater., vol. 322, pp. 3401-3409, 2010. View Article
[7] B. Belaabed, J. L. Wojkiewicz, S. Lamouri, N. El Kamchi, and T. Lasri, "Synthesis and characterization of hybrid conducting composites based on polyaniline/magnetite fillers with improved microwave absorption properties," J. Alloys Compd., vol. 527, pp. 137-144, 2012. View Article
[8] J. Pyun, "Nanocomposite Materials from Functional Polymers and Magnetic Colloids," Polym. Rev., vol. 47, pp. 231-263, 2007. View Article
[9] E. T. Kang, K. G. Neoh, and K. L. Tan, "Polyaniline: A polymer with many intrinsic redox states," Prog. Polym. Sci., vol. 23, pp. 277-324, 1998. View Article
[10] Y. S. Negi and P. V. Adhyapak, "Development in Polyaniline Conducting Polymers," J. Macromol. Sci. Part C Polym. Rev., vol. 42, pp. 35-53, 2002. View Article
[11] A.-H. Lu, E. L. Salabas, and F. Schüth, "Magnetic nanoparticles: synthesis, protection, functionalization, and application," Angew. Chemie Int. Ed., vol. 46, pp. 1222-1244, 2007. View Article
[12] M. A. Vergés, R. Costo, A. G. Roca, J. F. Marco, G. F. Goya, C. J. Serna, and M. P. Morales, "Uniform and water stable magnetite nanoparticles with diameters around the monodomain-multidomain limit," J. Phys. D. Appl. Phys., vol. 41, p. 134003, 2008. View Article
[13] Q. Xiao, X. Tan, L. Ji, and J. Xue, "Preparation and characterization of polyaniline/nano-Fe3O4 composites via a novel Pickering emulsion route," Synth. Met., vol. 157, pp. 784-791, 2007. View Article
[14] Y. Chung, S.-R. Yun, C.-W. Lee, N.-J. Jo, C.-H. Yo, and K.-S. Ryu, "Inorganic/Organic Nanocomposites of Polyaniline and Fe 3 O 4 with Hollow Cluster Structures using Polystyrene Template," Bull. Korean Chem. Soc., vol. 31, no. 7, pp. 2065-2068, 2010. View Article
[15] A. C. V. de Araújo, R. J. de Oliveira, S. Alves Jr, A. R. Rodrigues, F. L. A. Machado, F. A. O. Cabral, and W. M. de Azevedo, "Synthesis, characterization and magnetic properties of polyaniline-magnetite nanocomposites," Synth. Met., vol. 160, pp. 685-690, 2010. View Article
[16] S. E. Jacobo, J. C. Aphesteguy, R. Lopez Anton, N. N. Schegoleva, and G. V. Kurlyandskaya, "Influence of the preparation procedure on the properties of polyaniline based magnetic composites," Eur. Polym. J., vol. 43, no. 4, pp. 1333-1346, 2007. View Article
[17] J. C. Aphesteguy and S. E. Jacobo, "Synthesis of a soluble polyaniline - ferrite composite : magnetic and electric properties," J. Mater. Sci., vol. 42, pp. 7062-7068, 2007. View Article
[18] K. Basavaiah, Y. P. Kumar, and A. V. P. Rao, "A facile one-pot synthesis of polyaniline/magnetite nanocomposites by micelles-assisted method," Appl. Nanosci., vol. 3, pp. 409-415, 2013. View Article
[19] X. Ding, D. Han, Z. Wang, X. Xu, L. Niu, and Q. Zhang, "Micelle-assisted synthesis of polyaniline/magnetite nanorods by in situ self-assembly process," J. Colloid Interface Sci., vol. 320, pp. 341-345, 2008. View Article
[20] S. Radhakrishnan, C. R. K. Rao, and M. Vijayan, "Performance of Conducting Polyaniline-DBSA and Polyaniline-DBSA / Fe3O4 Composites as Electrode Materials for Aqueous Redox Supercapacitors," J. Appl. Polym. Sci., vol. 122, pp. 1510-1518, 2011. View Article
[21] H. R. Khorshidi, H. Eisazadeh, and A. R. Khesali, "Preparation and characterization of polyaniline containing Fe3O4 nanoparticles using sodium dodecylbenzenesulfonate as a surfactant," High Perform. Polym., vol. 23, pp. 125-131, 2011. View Article
[22] T. Sen, N. G. Shimpi, S. Mishra, and R. Sharma, "Polyaniline/γ-Fe2O3 nanocomposite for room temperature LPG sensing," Sensors Actuators B, vol. 190, pp. 120-126, 2014. View Article
[23] M. Rezvani, A. A. Asgharinezhad, H. Ebrahimzadeh, and N. Shekari, "A polyaniline-magnetite nanocomposite as an anion exchange sorbent for solid-phase extraction of chromium(VI) ions," Microchim. Acta, vol. 181, pp. 1887-1895, 2014. View Article
[24] F. Xu, L. Ma, Q. Huo, M. Gan, and J. Tang, "Microwave absorbing properties and structural design of microwave absorbers based on polyaniline and polyaniline/magnetite nanocomposite," J. Magn. Magn. Mater., vol. 374, pp. 311-316, 2015. View Article
[25] H. Gu, Y. Huang, X. Zhang, Q. Wang, J. Zhu, L. Shao, N. Haldolaarachchige, D. P. Young, S. Wei, and Z. Guo, "Magnetoresistive polyaniline-magnetite nanocomposites with negative dielectrical properties," Polymer, vol. 53, pp. 801-809, 2012. View Article
[26] T. J. B. Holland and S. A. . Redfern, "Unit cell refinement from powder diffraction data: the use of regression diagnostics," Mineral. Mag., vol. 61, pp. 65-77, 1997. View Article
[27] M. C. Arenas, E. Andablo, and V. M. Castaño, "Synthesis of Conducting Polyaniline Nanofibers from Single and Binary Dopant Agents," J. Nanosci. Nanotechnol., vol. 10, pp. 549-554, 2010. View Article
[28] T. Hino, T. Namiki, and N. Kuramoto, "Synthesis and characterization of novel conducting composites of polyaniline prepared in the presence of sodium dodecylsulfonate and several water soluble polymers," Synth. Met., vol. 156, pp. 1327-1332, 2006. View Article
[29] G. D. Prasanna, H. S. Jayanna, A. R. Lamani, and S. Dash, "Polyaniline/CoFe2O4 nanocomposites: A novel synthesis, characterization and magnetic properties," Synth. Met., vol. 161, pp. 2306-2311, 2011. View Article
[30] L. Li, J. Jiang, and F. Xu, "Synthesis and ferrimagnetic properties of novel Sm-substituted LiNi ferrite-polyaniline nanocomposite," Mater. Lett., vol. 61, pp. 1091-1096, 2007. View Article
[31] Z. Zhang and M. Wan, "Nanostructures of polyaniline composites containing nano-magnet," Synth. Met., vol. 132, pp. 205-212, 2003. View Article
[32] S. Khasim, S. C. Raghavendra, M. Revanasiddappa, K. C. Sajjan, M. Lakshmi, and M. Faisal, "Synthesis, characterization and magnetic properties of polyaniline/γ-Fe2O3 composites," Bull. Mater. Sci., vol. 34, pp. 1557-1561, 2011. View Article
[33] J. Jiang, L. Ai, and L.-C. Li, "Synthesis and magnetic performance of polyaniline/Mn-Zn ferrite nanocomposites with intrinsic conductivity," J. Mater. Sci., vol. 44, pp. 1024-1028, 2009. View Article
[34] W. Xue, H. Qiu, K. Fang, J. Li, J. Zhao, and M. Li, "Electrical and magnetic properties of the composite pellets containing DBSA-doped polyaniline and Fe nanoparticles," Synth. Met., vol. 156, pp. 833-837, 2006. View Article
[35] H. Gómez, M. K. Ram, F. Alvi, P. Villalba, E. (Lee) Stefanakos, and A. Kumar, "Graphene-conducting polymer nanocomposite as novel electrode for supercapacitors," J. Power Sources, vol. 196, pp. 4102-4108, 2011. View Article
[36] D. Thapa, V. R. Palkar, M. B. Kurup, and S. K. Malik, "Properties of magnetite nanoparticles synthesized through a novel chemical route," Mater. Lett., vol. 58, pp. 2692-2694, 2004. View Article
[37] R. H. Kodama, A. E. Berkowitz, E. J. McNiff, and S. Foner, "Surface spin disorder in ferrite nanoparticles (invited)," J. Appl. Phys., vol. 81, p. 5552, 1997. View Article