Volume 1, Issue 2, Year 2013 - Pages 17-25
DOI: 10.11159/ijtan.2013.002
Amphiphilic Oligomers Based On Diesters of Pyromellitic Acid for the Solubilization of Lipophilic Agents
Оlena Khomenko¹, Olga Budishevska¹, Аndriy Voronov², Оlena Kudina², Ihor Tarnavchyk¹² and, Stanislav Voronov¹
¹Lviv Polytechnic National University,
St. Bandery Str., 12, 79013, Lviv, Ukraine
²North Dakota State University, Dept. 2760,
P.O. Box 6050, Fargo, ND 58108-6050, USA,
budish@polynet.lviv.ua; andriy.voronov@ndsu.edu
Abstract - New amphiphilic oligomeric surfactants, diesters of pyromellitic acid (DEPA), were synthesized from pyromellitic dianhidryde, polyethylene glycol methyl ethers of different chain lengths, and alkanols/cholesterol. The synthesis resulted in amphiphilic oligomers containing hydrophilic (polyethylene glycol methyl ether, MPEG) and hydrophobic (residues of alkanol/cholesterol) fragments with a defined length. We demonstrated that DEPA oligomers form micelles in an aqueous solution, and that the surface activity of DEPA depends on the nature and length of its hydrophilic and hydrophobic constituents (the hydrophilic-lipophilic balance of DEPA). Above their critical micelle concentration, the DEPA oligomers solubilize water-insoluble substances (including the lipophilic drug, curcumin), which confirms their potential for improving the solubility of poorly water-soluble (lipophilic) agents (drugs), for example in drug delivery systems.
Keywords: Surface active diesters of pyromellitic acid, solubilization of lipophilic agents.
© Copyright 2013 Authors - This is an Open Access article published under the Creative Commons Attribution License terms. Unrestricted use, distribution, and reproduction in any medium are permitted, provided the original work is properly cited.
Date Received: 2013-10-16
Date Accepted: 2013-11-08
Date Published: 2013-11-18
1. Introduction
The development of a drug delivery platform based on polymer micelles for transporting hydrophobic (water-insoluble) drugs to pathological cells is a novel approach that has the potential to address the limitations of traditional drug delivery systems [1]. Recent studies resulted in the development of a large number of chemotherapeutic drugs and other therapeutic agents for the treatment of a wide range of cancer, cardiovascular and inflammatory diseases. However, the therapeutic potential of these agents is limited, primarily due to their poor solubility in aqueous media. To overcome this problem, significant research efforts have been recently made to develop novel nanotherapeutic modalities, including amphiphilic molecular assemblies: micelles, vesicles, and liposomes. These nanomodalities possess physico-chemical characteristics that can potentially fulfill the requirements of efficient drug delivery by providing colloidal dissolution of lipophilic agents in the micellar hydrophobic interior, while stabilizing the agent in aqueous media through the hydrophilic outer part of the micelles.
In our previous study, we demonstrated the synthesis of amphiphilic comb-like macromolecules through the polymer-analogous reactions of α-olefin - maleic anhydride copolymers with MPEG to obtain alternating hydrophilic (MPEG) and lipophilic (alkyl) side chains, in a polymeric surfactant backbone [2]. In aqueous solution, these polymeric surfactants self-assemble into micellar structures that can solubilize poorly water-soluble substances (lipophilic dyes, aromatic hydrocarbons), thus improving their solubility in water.
In this study, new oligomeric surfactants, the amphiphilic diesters of pyromellitic acid (DEPA), were synthesized from pyromellitic dianhidryde, polyethylene glycol methyl ethers with different chain lengths, and alkanols/cholesterol (Figure 1). The synthesis resulted in amphiphilic surface active oligomers, including diblock oligomers, containing hydrophilic (polyethylene glycol methyl ethers, MPEG) and hydrophobic (residues of alkanol/cholesterol) fragments with a defined length. Our expectation is that the inner (solubilizing) part of the oligomeric micelles consists of the DEPA hydrophobic constituents, while the micellar stabilizing exterior is from MPEG chains, which are known to provide micellar stability and longevity in the bloodstream. Additional control over the DEPA micellization is provided by the variation in hydrophilic (different MPEG lengths) and hydrophobic (butyl, octyl, cetyl, cholesteryl moieties) fragments in the DEPA molecule during the synthesis. In this way, the variation of the hydrophilic-lipophilic balance (HLB) of DEPA was achieved to considerably influence surface activity, and broaden the physico-chemical properties of the DEPA micelles. Additionally, it is expected that a controlled number of ester bonds in the DEPA molecules can provide biodegradability to the molecules of the synthesized oligomer surfactants. Choosing a cholesterol, an essential structural component of the cell membrane, as one of the DEPA hydrophobic fragments, targets a potentially enhanced interaction between the oligomeric micelles and the biological membranes.
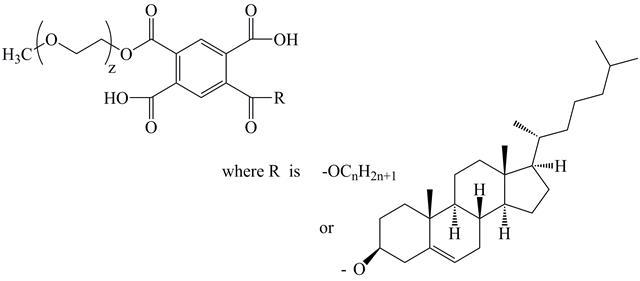
Recently, several studies have focused on the development of amphiphilic surfactants capable of solubilizing and delivering the curcumin (a phytochemical agent that occurs naturally in plants) as an antitumor agent, and thereby increasing its bioavailability and efficacy in cancer treatment [3,4]. The clinical development of curcumin is, however, still hindered due to the drug's insolubility in water, restricting the use of the curcumin. It is our expectation that DEPA oligomeric surfactants can be considered as novel and promising biomaterials in solubilizing water-insoluble drugs, including curcumin, for further improvement in the administration of lipophilic agents.
2. Methods and Materials
2.1. Materials
Pyromellitic dianhydride (PMDA, 97%) was purchased from Sigma Aldrich and used as received. Polyethylene glycols methyl esters (Aldrich, ≥98%) with molecular weights of 350 g/mol (MPEG350), 550 g/mol (MPEG550), and 750 g/mol (MPEG750) and cholesterol (Chol) (Aldrich) were purified by dissolving them in benzene (30% solution) followed by a distillation of the benzene-water azeotropic mixture at 393K. Primary aliphatic alcohols (PAA) (Sigma Aldrich, ≥98%), 1-butanol (But) and 1-octanol (Oct), were purified by boiling them with CaO for 4 hours and 2 days, respectively, and subsequent distillation over magnesium chips. Cetyl alcohol (Cet) (Aldrich) was used without purification. Dimethylformamide (DMFA) (Sigma Aldrich, anhydrous, 99.8%) was used as received. Triethylamine (TEA) (Sigma Aldrich, ≥99%) was purified by simple vacuum distillation. Curcumin (Overseal Natural Ingredients Ltd), 4-methyl-2-pentanone (Sigma Aldrich, ACS reagent, ≥98.5%), acetone-d6 and chloroform-d (Aldrich, 99.9 atom % D) were used without purification. A fluorescent probe, pyrene (Alfa Aesar, 98%) was used as received.
2.2. Synthesis of DEPAs
Oligomeric diesters of pyromellitic acid were synthesized using a two-step esterification of PMDA, with primary aliphatic alcohols (Alc)/Cholesterol (Chol) and MPEG. The synthesis was carried out in DMFA in the presence of a catalyst TEA (0.02 mol/l), at 353K in an argon atmosphere. For the synthesis of Alc-PMDA-MPEG, 1M solution of aliphatic alcohol and PMDA in DMFA was stirred at a 1:1 ratio during 24 hours. Afterwards, an equimolar quantity of MPEG was added to the mixture and stirred for another 24 hours during the 2nd reaction stage. For the synthesis of Chol-PMDA-MPEG, 1M solutions of MPEG and PMDA in DMFA were first stirred at a 1:1 ratio for 24 hours. Afterwards, an equimolar quantity of cholesterol was added to the mixture and stirred for another 24 hours during the 2nd reaction stage. Reaction conversion (97-99% at both stages) was controlled by the content of carboxyl groups, determined by pH-metric titration of 0.6 mL reaction mixture (diluted in excess DMFA) by 0.1 N aqueous sodium hydroxide.
After the synthesis, DMFA was distilled from the reaction mixture using a rotary evaporator. Alc-PMDA-MPEG esters were purified by multiple precipitation from the acetone solution into hexane, and washed using 4-methyl-2-pentanone to remove any side products, as well as MPEG and PMDA residues (final product yield 75-85%). Chol-PMDA-MPEG was purified by filtering the acetone solution to remove the cholesteryl pyromellititate esters (insoluble in acetone) and by using multiple precipitation from acetone into hexane to remove the residual cholesterol. The precipitate was dried, dissolved in aqueous sodium carbonate solution, and precipitated by adding a 5% HCl solution and excess of saturated NaCl solution. (The final product yield was 50-60%). Purified DEPAs were dried at 328K.
2.3. FTIR Spectroscopy
The FTIR spectra of synthesized oligomers were recorded in a thin layer deposited from the benzene solution on a potassium bromide tablet, using a Thermo Scientific Nicolet Fourier Transform Infrared Spectrometer, in the range of 400-4000 cm-1 with compensation of atmospheric CO2 and H2O.
2.4. NMR Spectroscopy
NMR spectra of oligomers were recorded in acetone-d6 and chloroform-d (Chol-PMDA-MPEG550) solutions using a 500 MHz Varian Inova spectrometer.
2.5. Surface Tension to Determine CMC of DEPAs
The surface tension measurements were performed by drop shape analysis on pendant drops with a Contact Angle/Surface Tension Analyzer, First Ten Angstroms (FTÅ125) to determine the CMC of the DEPAs. Measurements were carried out at room temperature for the oligomer solutions of various concentrations (10−7% – 1% wt). Aqueous DEPA solutions were prepared by dissolving the DEPA in a Millipore water (18W) and further adjusting the pH using a 15% aqueous sodium carbonate solution. All glassware was washed in a 1 N NaOH bath and thoroughly rinsed with Millipore water before use.
2.6. Fluorescence Spectroscopy to Determine CMC of DEPAs
Alternatively, the critical micelle concentration of DEPAs was measured using the solubilization of a fluorescent probe, pyrene, using a previously reported method [5, 6]. The spectra were taken using a Fluoromax-3 Fluorescence Spectrometer (Jobin Yvon Horiba) with 90° geometry, and a slit opening of 0.5 nm. For fluorescence excitation spectra, λem = 390 nm was chosen. Spectra were accumulated with an integration time of 0.5 nm/s. Critical micelle concentration values were determined after fitting the semi-logarithmic plots of intensity ratio I336.5/I333 versus log concentration to the sigmoidal curve.
2.7. Curcumin Solubilization in DEPA Micelles
To determine curcumin solubilization, a sample of curcumin powder was mixed with different oligomer concentration solutions in a phosphate buffer (pH 6.58), and stirred at room temperature for 48 hours. Excessive curcumin was filtered. The UV-vis spectra of curcumin-solubilized oligomer solutions were recorded using Varian Cary 5000 at 293K. The concentration of solubilized curcumin was determined from the absorption spectra at characteristic 470 nm wavelength, using a calibration curve. The calibration curves were built after recording the UV−vis spectra of a set of curcumin solutions of known concentrations in the 1-octanol.
2.8 Solubilization of Non-Polar Solvents in DEPA Micelles
To study the solubilization of hexane/benzene in DEPA micelles, 0.01 ml of hexane/benzene was gradually added, every 2 days, to 20 mL of aqueous oligomer solution. The optical density (D) of the DEPA aqueous solutions were measured using the Photoelectric Colorimeter K 77 (λ = 535 nm) every two days, before adding a new portion of hexane/benzene. The procedure was repeated 10-15 times until the solution became turbid. The experimental values of D were plotted versus the volume of hexane/benzene added and the solubilization amount was determined as a slope change.
3. Results and Discussion
3.1 DEPA Synthesis and Characterization
Figure 2A, B shows the chemical structures of the diesters of pyromellitic acid (A- Alc-PMDA-MPEG, B -Chol-PMDA-MPEG) that were synthesized and used in this study for the formation of micelles and the further solubilization of lipophilic agents.
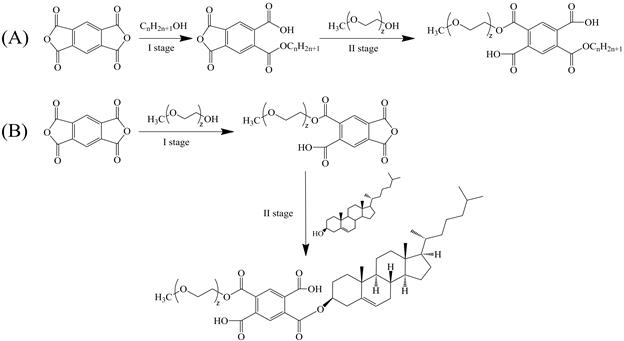
The chemical structure of the DEPAs was confirmed using FT-IR and 1H NMR spectroscopy (Figures 3-5). Corresponding to methyl and methylene groups, the characteristic bands for aromatic rings, ester groups and ether fragments on the FTIR spectra (Figure 3, Tables 1,2.) confirmed the chemical structure of the Alc/Chol -PMDA-MPEG oligomers.
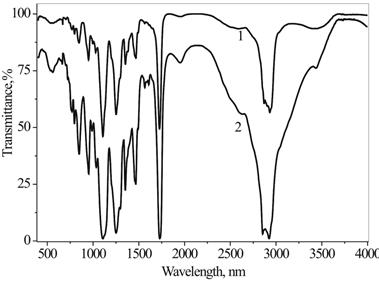
Table 1. Characteristic bands on FTIR spectra of Alc-PMDA -MPEG oligomers.
Wavenumber, cm-1 |
Group |
3426 ÷ 3438 |
COOH group near aromatic ring |
2927 ÷2921 |
СН3 (alkyl) |
2854 ÷2869 |
СН2 (alkyl) |
1957¸1950 |
Overtone band for substituted aromatic ring |
1729 ÷1727 |
С=О (ester) |
1612 ÷1616 |
Aromatic ring |
1467 ÷1456 |
СН2 (alkyl) |
1253 ÷1249 |
С-О- (ester) |
1106 ÷1105 |
С-О-С (ether) |
953¸951 |
С-Н (aromatic) (CH out-of-plane bending) |
760 ÷750 |
СН2 (alkyl) |
The chemical structure and composition of the synthesized products was confirmed using 1H NMR spectroscopy. 1H NMR spectra of Cet-PMDA-MPEG550 and Chol-PMDA-MPEG550, along with chemical shifts of protons, are represented in Figures 4 and 5 with Table 3 showing good agreement with the published data [7,8].
Table 2. Characteristic bands on FTIR spectra of Chol-PMDA –MPEG550.
Wavenumber, cm-1 |
Group |
3400 ÷ 3500 |
COOH group near aromatic ring |
2931 |
СН3 (alkyl) |
2870 |
СН2 (alkyl) |
1726 |
С=О (ester) |
1468 |
СН2 (alkyl) |
1354 |
alkyl |
1252 |
ОС-О- (ester) |
1107 |
С-О-С (ether) |
841 |
С-Н (aromatic) (CH out-of-plane bending) |
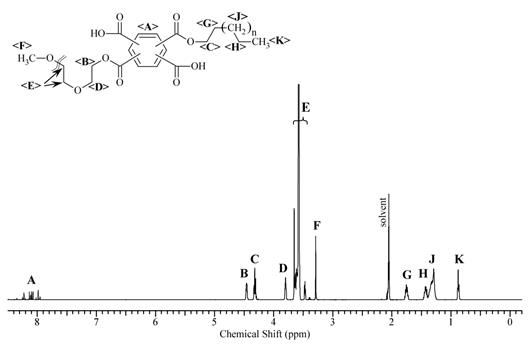
As can be seen from the data, the protons' signals for the MPEG550 fragment (E) show a chemical shift of 3.57 ppm for all synthesized diesters. An integral for the protons G (methylene groups of the alkyl fragment) was assigned as 2.0 (Table 3) and divided by two (since there are two protons in the methylene group).
The MPEG550 fragment contains approximately 44 protons, thus the integral for E protons should be divided by 44. The ratio of G/2 to E/44 for the synthesized products is : for But-PMDA -MPEG550: [G/2]:[E/44] = [2,00 / 2]: [44.34 / 44] = 1.00, for Oct-PMDA-MPEG550: [G/2]:[E/44]= [2,00 / 2]: [46,50 / 44] = 0.94; within Cet-PMDA-MPEG550: [G/2]:[E/44]= [2,06 / 2]: [52.0 / 44] = 0.87. Hence, the ratio of the proton group's intensity (i.e. ratio of protons' intensities for the hydrophilic and lipophilic chains) is in good agreement with the theoretical values, confirming the synthesis and further successful purification of the DEPA oligomers. To this end, the acylation reaction of aliphatic alcohols and polyethylene glycol methyl ethers by PMDA can be employed for the synthesis of new amphiphilic oligomers based on diesters of pyromellitic acid.
Table 3. H NMR characterization of oligomersa.
# |
Assignmenta |
Chemical Shift, ppm |
Number of protons |
Sample Integral |
||
But-PMDA-MPEG550 |
Oct-PMDA-MPEG550 |
Cet-PMDA-MPEG550 |
||||
1 |
K |
0.85-0.95 |
3 |
2.48 |
2.30 |
2.48 |
2 |
H |
1.38-1.47 |
2 |
2.00 |
10.46 |
35.88 |
3 |
J |
1.27-1.37 |
- / 8 / 24b |
- |
||
4 |
G |
1.70-1.78 |
2 |
2.00 |
2.00 |
2.06 |
5 |
C |
4.29-4.33 |
2 |
1.94 |
2.34 |
2.84 |
6 |
A |
7.90-8.50 |
2 |
1.28 |
1.62 |
1.40 |
7 |
B |
4.40-4.45 |
2 |
1.70 |
1.92 |
2.04 |
8 |
D |
3.80 |
2 |
46.72 |
47.12 |
51.98 |
9 |
E |
3.45-3.70 |
44 |
a Assignment of protons corresponds to Figure 4.
b Value corresponds to the composition of sample: But-PMDA-MPEG550, Octy-PMDA-MPEG550 or Cet-PMDA-MPEG550.
Signals for the methyl groups' (chemical shift 0,85-0,86 ppm) and methylene groups' (1,02-1,99 ppm) protons, corresponding to the signals of protons in alkyl and cyclic fragments of cholesterol, respectively, were observed in the Chol-PMDA-MPEG550 NMR spectrum (Figure 5). A group of signals with a chemical shift in a range 3.63 ÷ 3.76 ppm corresponds to the methylene groups' protons in the MPEG550 fragments, while signals with a chemical shift of 3.37 ppm correspond to the methyl protons of the same fragment. Signal with a chemical shift of 4.46 ppm correspond to the protons of the PEG methylene groups (D) in α position. Multiplet at 4.86 ppm depicts cholesterol fragment proton in the α position to the carboxylate group (C). The signal at 5.40 ppm corresponds to the proton at the carbon atoms forming the double bond in cholesterol (B). Signals in the range 7,99÷8,23 ppm correspond to the protons of the aromatic fragment. The assignment of chemical shifts is confirmed by a ratio of the integrals for the MPEG and the cholesterol fragments: (I MPEG:45)/(I Chol/44) ≈ (49:45) / (49.18:44) ≈ 0 99, where 45 and 44 are the number of protons in MPEG550 and the cholesterol fragments respectively. Moreover, the integral for one proton C is 1.07, two protons D is 1.94, two protons A is 1.96, further confirming the structure of the product.
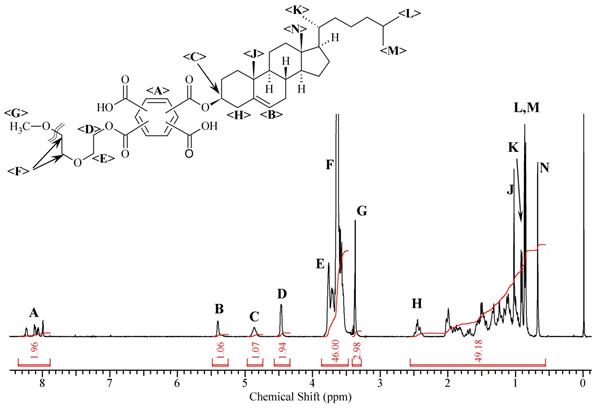
3.2 Surface Activity of DEPA Oligomers
Amphiphilic behavior of the synthesized Alc-PMDA-MPEG and Chol-PMDA-MPEG oligomers is expected from having lipophilic alkyl/cholesterol fragments and hydrophilic MPEG fragments, simultaneously in the DEPA chemical structure. Broad surface activity for synthesized DEPAs was targeted to achieve a varying capacity of micelles in the solubilization of lipophilic agents in water. To this end, the HLB of the DEPAs was varied by changing the length of the hydrophilic and hydrophobic fragments in the oligomer molecule between 6.1 and 14.2, as calculated [8]. To confirm the formation of micelles from DEPAs in the aqueous solution, CMC values were determined by using surface tension measurements and the solubilization of pyrene. Molecular weight, hydrophilic lipophilic balance and critical micelle concentration of DEPAs (determined by using surface tension measurements) are shown in Table 4.
Pyrene is a well-known fluorescent probe for studying the association behavior of amphiphilic polymers [5,6]. Depending on the environment of the pyrene, a red shift of the absorption band, with enhanced excitation intensity, was observed due to the migration of the probe from the hydrophilic to the hydrophobic region of the polymer micelles. In our experiments, pyrene excitation spectra were monitored in the wavelength range of 300−360 nm. From the pyrene excitation spectra, the intensity ratios I336.5/I333 were plotted as a function of oligomer concentration (Figure 6). A red shift of the fluorescence excitation spectra from 333 to 336.5 nm, with an increasing DEPA concentration in aqueous solution, indicates the solubilization of pyrene within the micellar hydrophobic environment, and the transfer of pyrene molecules from the water to the micelles. The sharp increase in the intensity ratio corresponds to the critical micelle concentration for each DEPA composition.
Table 4. Chemical structure and characteristics of DEPAs used in this study.
Lipophilic fragment |
Hyprophilic MPEG, MW |
Oligomer, MW |
HLB |
CMC, mmol/L |
|
|
|
||||
Oct |
-С8Н17 |
350 |
715 |
11, 2 |
13.6 |
Oct |
-С8Н17 |
550 |
915 |
12.7 |
11.7 |
Cet |
-С16Н33 |
550 |
1027 |
8.9 |
2.0 |
Chol |
-C27H45O |
550 |
1188 |
6.1 |
0.8 |
Oct |
-С8Н17 |
750 |
1115 |
14.2 |
11.1 |
Cet |
-С16Н33 |
750 |
1227 |
10.4 |
2.0 |
CMC values correlate with the chemical structure and HLB of the DEPAs. Changing the length of both the hydrophobic and hydrophilic fragments in the DEPA molecules results in a significant difference in recorded CMC values, both when using surface tension measurements and through the solubilization of the fluorescence probe.
Determined using surface tension measurements CMC generally increases with an increasing HLB, and shows strong dependence on the length and nature of the DEPA hydrophobic fragment. The highest surface activity was recorded for the Chol-PMDA-MPEG550 oligomer. Three oligomers based on MPEG550 and different hydrophobic fragments, were chosen for CMC measurements using fluorescence probe solubilization. Figure 6 shows that this method gives, in general, lower values for CMC, but with the same trend, indicating higher surface activity of more hydrophobic oligomer and lower CMC. The CMC for the oligomers varies between 1 x 10-3 mol/L and 4.8 x 10-2 mol/l.
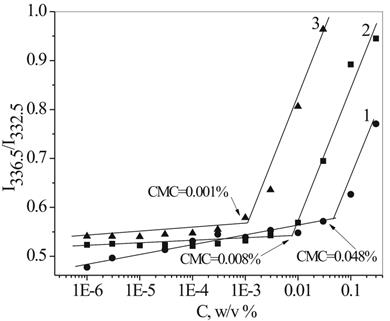
This observation clearly indicates that synthesized DEPA molecules are surface active and form micelles in aqueous solution due to the aggregation of single oligomeric molecules in assemblies at different concentration, depending on the HLB (chemical structure) of the oligomers.
3.3. Solubilization of Lipophilic Agents using DEPA Micelles
The ability of DEPA micelles to solubilize otherwise insoluble substances in water was demonstrated using two non-polar solvents, hexane and benzene, both immiscible with water. In the presence of DEPA micelles, both non-polar solvent molecules were immediately extracted into an aqueous phase, that was accompanied by clear changes in the DEPA aqueous solution turbidity. Using spectrophotometry, it was demonstrated that hexane and benzene molecules can be physically incorporated within the hydrophobic interior of theDEPA micelle in aqueous solutions in significant quantities (6-8 moles of solvent/mol DEPA).
Having established the DEPA micelles' ability to solubilize insoluble substances in water, we chose four oligomeric surfactants, with different surface activity, to demonstrate the capability of their micellar solutions to be loaded with the molecules of a lipophilic drug, poorly water-soluble curcumin. All chosen DEPAs demonstrated that curcumin can be successfully solubilized by the DEPA micellar assemblies (Figure 7). The amount of the solubilized drug differs significantly for different DEPA surfactants, showing a general tendency to increase in relation to the increasing length of the hydrophobic fragments.
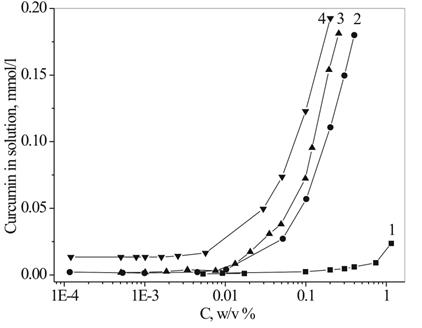
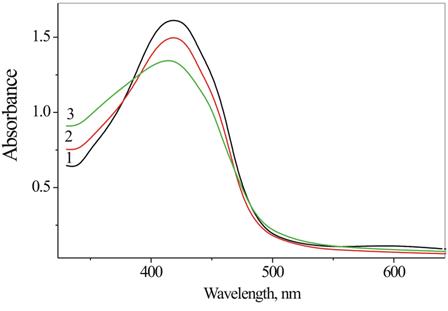
In addition, the DEPA micelles stabilize curcumin molecules in an aqueous medium and prevent drug decomposition. It is known that curcumin is stable at an acidic pH, but is not stable at neutral and basic pH. In these conditions it decomposes to (4′- hydroxy-3′-methoxyphenyl)-2,4-dioxo-5-hexanal, ferulic acid, and feruloyl methane. [9] It has been demonstrated that more than 90% of the curcumin rapidly decomposes within 30 min of placement in phosphate buffered saline at pH 7.2.
In this work, the stability of the curcumin loaded in DEPA micellar assemblies was monitored by UV−vis spectroscopy. The data show that the change in absorbance of the curcumin-loaded DEPA micelles is small, confirming a good stability of micellar curcumin. In particular, very low curcumin decomposition degree was observed for micellar formulation based on Cet-PMDA-MPEG550 during 560 hours (Figure 8).
4. Conclusions
A new class of amphiphilic oligomers, surface active diesters of pyromellitic acid, was synthesized using pyromellitic dianhidryde, polyethylene glycol methyl ethers of different chain lengths, and alkanols/cholesterol. The synthesis resulted in amphiphilic oligomers containing hydrophilic (polyethylene glycol methyl ethers, MPEG) and hydrophobic (residues of alkanol/cholesterol) fragments of defined lengths. We demonstrated that DEPA oligomers form micelles in an aqueous solution, and the surface activity of DEPA depends on the nature and length of the hydrophilic and hydrophobic constituents (the hydrophilic-lipophilic balance of DEPA). Above their critical micelle concentration, the DEPA oligomers solubilize water-insoluble substances (including a lipophilic drug, curcumin), which confirms their potential in improving the solubility of poorly water-soluble (lipophilic) agents (drugs), for example in drug delivery systems. Additionally, it was demonstrated that loading in DEPA micelles significantly increases the stability of poorly water-soluble drug, curcumin at neutral pH in aqueous media. It is expected that DEPA micellar rnanomodalities possess physico-chemical characteristics that can potentially fulfill the requirements (including size distribution, chemical structure, "capacity" in terms of the encapsulated cargo) of efficient drug delivery by providing colloidal dissolution of lipophilic agents in the micellar hydrophobic interior, while stabilizing the agent in aqueous media through the hydrophilic outer part of the micelles.
References
[1] R. Sinha, G. J. Kim, S. Nie and D. M. Shin, "Nanotechnology in cancer therapeutics: bioconjudgated nanoparticles for drug delivery" 2006, Molecular Cancer Therapeutics, 5 1909-17. View Article
[2] O. Kudina, O. Budishevska, A. Voronov, A. Kohut, O. Khomenko, S. Voronov, "Synthesis of New Amphiphilic Comb-Like Copolymers Based on Maleic Anhydride and α-Olefins" 2010, Journal of Macromolecular Symposia, 298, 79–84. View Article
[3] M. H. M. Leung, H. Colangelo, T.W. Kee, "Encapsulation of Curcumin in Cationic Micelles Supporesses Alkaline Hydrolysis", 2008, Langmuir 24 5672-75. View Article
[4] S. Bisht, G. Feldmann, S. Soni, R. Ravi, C. Karikar, A. Maitra and A. Maitra, "Polymeric nanoparticle-encapuslated curcumin ("nanocurcumin"): a novel strategy for human cancer therapy", 2007, Journal of Nanobiotechnology 5 3. View Article
[5] C. Schmitz, A. Mourran, H. Keul and M. Möller, "Synthesis and association behavior of linear block copolymers with difference microstructures but the same composition", 2008 Macromolecular Chemistry and Physics, 209 1859–71 View Article
[6] M. Wilhelm, C. L. Zhao, Y. Wang, R. Xu, M. A. Winnik, J. L. Mura, G. Riess and M. D. Croucher, "Poly(styrene-ethylene oxide) block copolymer micelle formation in water: a fluorescence probe study", 1991 Macromolecules 24 1033–40 View Article
[7] J. T. Davies and E. K. Rideal, 1961 Interfacial Phenomena (New York: Academic Press)
[8] D. W. Brown, A. J. Floyd, and M. J. Sainsbury 1988 Organic Spectroscopy (New York: John Wiley Sons)
[9] A. Kumar, A. Ahuja, J. Ali and S. Baboota, "Conundrum and therapeutic potential of curcumin in drug delivery", 2010 Critical Reviews in Therapeutic Drug Carrier Systems, 27 279– 312. View Article