Volume 1, Issue 1, Year 2012 - Pages 111-116
DOI: 10.11159/ijtan.2012.017
Effect of Size and Surface Ligands of Silver (Ag) Nanoparticles on Waterborne Bacteria
Toqeer Ahmed¹*, Saba Imdad¹, Sumaira Ashraf², Noor Mohammad Butt³
¹ Preston Institute of Nano Science & Technology (PINSAT), Preston University, Sector H-8/4, Islamabad, 44000 - Pakistan.
²Department of Chemistry, School of Science & Engineering (SSE), Lahore University of Management Sciences (LUMS), Lahore Cantt-54792, Pakistan.
³3 Preston Institute of Nano Science & Technology (PINSAT), Preston University, H-8/4,
Islamabad, 44000 - Pakistan.
hellotoqeer04@yahoo.com; elegantsvelte22@gmail.com; sumaira.ashraf@lums.edu.pk; nmbutt36@yahoo.com
Abstract - Clean drinking water is a matter of concern for all countries, more so for many developing countries and consequently various methods of producing clean drinking water are being practiced for provision of such water to the consumers. At the same time an attempt is always made to adopt any new technique or technology to use it for commercially feasible production of such water. In recent years, with the advent of fast developing technologies, nanotechnology has also come into operation for economic and commercial production of clean drinking water. With a view to produce clean drinking water free from pathogens by the use of nanotechnology, we have investigated the antibacterial properties of silver (Ag) nanoparticles against waterborne bacteria in the public water supply sources in Islamabad, the capital of Pakistan. Water samples from over 50 water supply sources were collected and bacterial strains were identified by API20E method. Different silver nanoparticles were tested against 8 different bacterial isolates at concentrations varying from 26 to about 77 µg/mL. Nanoparticles of size ranging from 3-8 nm prepared by casein stabilization method showed the best activity against all isolated strains, E. coli being the most effectively inhibited with an average 14.9 mm zone of inhibition. C. sakazakii (12.6 mm) was most sensitive to citrate stabilized particles, followed by E. coli (12.3 mm), K. pneumoniae (12 mm) and P. aeruginosa (11.6 mm). These results provide a useful picture for the development of water filters incorporated with silver nanoparticles. We believe that this study is important for the public, particularly in the developing countries and also for others working in the area of antibacterial effects of nanoparticles.
Keywords: Silver nanoparticles, Waterborne bacteria, Drinking water, Antibacterial effect, Surface ligands
© Copyright 2012 Authors - This is an Open Access article published under the Creative Commons Attribution License terms. Unrestricted use, distribution, and reproduction in any medium are permitted, provided the original work is properly cited.
1. Introduction
Among the many different types of metallic and metal oxide nanoparticle (NPs), Silver (Ag) NPs have proven to be the most effective against bacteria, viruses, and other eukaryotic microorganisms (Liu et al., 2009; Chamundeeswari et al., 2010; Huang et al., 2009; Sharma et al., 2008). Antibacterial activity of Ag is very well known from history. Ag NPs attack the respiratory chain and cell division that finally lead to cell death, while concomitantly releasing silver ions that enhance bactericidal activity (Klasen 2000).
Several investigators have assessed the biocidal efficacy of silver nanoparticles. (Baker et al., 2005; Panacek et al., 2006; Kim et al., 2007; Shrivastava et al., 2007; Li et al., 2010)Silver significantly reduces waterborne pathogens like Pseudomonas aeruginosa and Aeromonas hydrophila by inactivating them. It can also be used as a secondary disinfectant (Silvestry-Rodriguez et al., 2007). Kim et al. (2007) reported the effect of silver nanoparticles against yeast, Escherichia coli, and Staphylococcus aureus. They reported that Ag nanoparticles can be used as effective growth inhibitors against various microorganisms, which makes it capable of being applied in diverse medical devices and systems for antimicrobial control. Elechiguerra et al. (2005) studied the size dependent effect of silver nanoparticles on HIV-1 virus. They reported that silver nano particles inhibit the binding of virus to the host cells in vitro. Sondi and Salopek-Sondi (2004), studied the antibacterial effect of silver nano particles against Escherichia coli. Scanning and transmission electron microscopy (SEM and TEM) were used to study the biocidal action of this nano scale material. Their results confirmed that the treated Escherichia coli cells were damaged, showing formation of "pits" in the cell wall of the bacteria. They reported that these nontoxic nano materials, which can be prepared in a simple and cost-effective manner, may be suitable for the formulation of new types of bactericidal materials. In another study, the bactericidal effect of silver nanoparticles obtained by a novel electrochemical method on Escherichia coli, Staphylococcus aureus, Aspergillus niger and Penicillium phoeniceum cultures were studied. The silver nanoparticles show a pronounced antibacterial/antifungal effect (Khaydarov et al., 2009). Parameswari et al. (2010) compared the antimicrobial activity of silver nano particles and AgNO3 against E. coli in terms of growth rate, time dependency and zone of inhibition. Ag nano particles created a zone of inhibition of 1.7 cm as compared to 0.8 cm by AgNO3, thus proving Ag nanoparticles to be the efficient candidate for antimicrobial activity. Similarly, another study by Morones et al. (2005) on bactericidal effect of silver nanoparticles against gram negative bacteria showed that the effect is size dependent and the most effective range of nanoparticle sizes that provided direct interaction with the bacteria had a diameter of ~1–10 nm amongst the tested range of 1-100 nm.
Silver nanoparticles are highly active and show better biocidal results against Gram negative bacteria such as E. coli (Tiwari and Behari, 2009) as compared to Gram positive bacteria and the effects are dose dependent (Madigan and Martinko, 2005). The antimicrobial activity of Ag NPs is inversely dependent on size (Raimond et al., 2005; Sondi and Salopek-Sondi, 2004). Pal et al. (2007) reported that antibacterial activity of silver nanoparticles is also dependent upon the shape of the nanoparticles. There is another report showing the use of fullerenes and carbon nanotubes as antimicrobial agents (Kang et al., 2007; Badireddy et al., 2007). In this study, antibacterial activity of Ag nanoparticles (Ag NPs) was tested against different Gram positive and Gram negative waterborne bacterial strains which were isolated and identified from water samples taken from different water supply sources of Islamabad working under Capital Development Authority (CDA).
2. Material and Methods
2.1. Synthesis of Ag Nanoparticle
Ag nanoparticles of three particles sizes (S1= 3-8nm, S2= 4-8nm and S3 = 5-9 nm) were prepared for the present investigation by following methods.
2.1.1. Synthesis of Casein (protein) Stabilized Sliver Nanoparticles (S1)
In a typical experiment to synthesize casein (protein) stabilized silver nanoparticles, a given volume of a warm aqueous suspension of casein, set at a desired pH (7-8), was added quickly to a boiling aqueous solution of AgNO3 (25 mL, 1 mM) under vigorous stirring. The color of the reaction mixture gradually changed from colorless to brown within 5 – 10 minutes. The reflux was continued for one hour to ensure complete reaction resulting in the formation of a brownish silver nanoparticles suspension. The nanoparticle suspension was then filter purified using centrifuge filters (Amicon® Ultra Centrifugal Filters by Millipore Corporation) having a molecular cut off value of 100kDa to remove excess casein and other impurities and then stored at room temperature for further analysis and use in subsequent experiments. Casein (molecular weight 75–100 kDa) used in this work was of Hammerstein grade purchased from MP Biomedicals. All solutions of casein were prepared in 50 mM Tris buffer and pH adjusted using 1 M NaOH/H2SO4. Ultrapure water with a resistivity of 18.2 MΩ. cm was used as the solvent in all preparations. The approximate size of these silver nanoparticles was found to be ca. 3-8 nm. FTIR spectroscopy confirmed that the hydrophobic part and amide functionality of casein is involved in the stabilization of silver nanoparticles (Ashraf et al., 2013).
2.1.2. Synthesis of Citrate Stabilized Silver Nanoparticles (S2)
This method is with slight modifications in the classical citrate reduction method by Turkevich et al. (1951) for nanoparticle synthesis. To an aqueous solution of 1mM AgNO3, 1mM tri-sodium citrate solution was added and stirred for 2 hours. After 2 hours 5 mL NaBH4 solution (0.8 mg/l mL) was added during stirring at room temperature. The reaction was allowed to complete for 5 hours. The particle solution was filter purified to remove access ligand and other impurities using centrifuge filters (Amicon® Ultra Centrifugal Filters by Millipore Corporation) having a molecular weight cut off value of 30 KDa, and then stored at room temperature for further analysis and use in subsequent experiments. The approximate size of these silver nanoparticles was found to be ca. 4-8 nm and pH was between 7 to 8.
2.1.3. Synthesis of a Polyhexamethylene biguanide (PHMB) Stabilized Silver Nanoparticles (S3)
In a typical experiment to prepare polymer (PHMB) functionalized silver nanoparticles, a given volume (15 mL, 20 μg/mL) of PHMB solution, was added to an aqueous solution of 1mM AgNO3 under vigorous stirring. The reaction mixture was allowed to stir vigorously for 2 hours to let polymer form a complex with silver. Freshly prepared aqueous solution of sodium borohydride (5 mL, 0.8 mg/mL) was then added quickly to the polymer-Ag solution and the reaction was allowed to complete for 5 hours. The yellow/brownish suspension of sliver nanoparticles was then filter purified to remove excess polymer and other impurities using centrifuge filters (Amicon® Ultra Centrifugal Filters by Millipore Corporation) having a molecular weight cut off value of 30 KDa, and then stored at room temperature for further analysis and use in subsequent experiments. PHMB (20% w/v) was purchased from Arch Chemicals (Norwalk, CT, USA). The approximate size of these silver nanoparticles was found to be ca. 5-9 nm. FTIR spectroscopy indicated the interaction of imine group of PHMB with nanoparticles confirming its functionalization with PHMB (Ashraf et al., 2012).
2.2. Characterization of Silver Nanoparticles
The silver nanoparticles were characterized using UV-visible spectroscopy in the range of 300 to 800 nm. All the three preparations have a clear surface plasmon resonance band. The particles produced by borohydride reduction in the presence of sodium citrate seem relatively more uniform as indicated by the narrow absorption band.
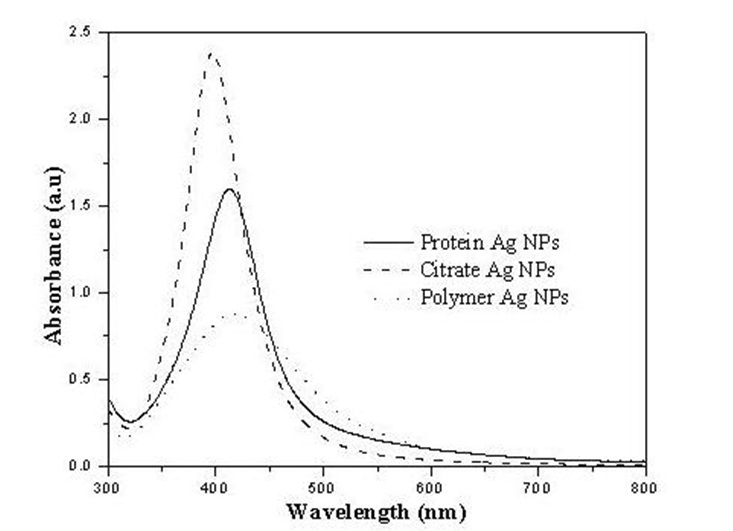
Three samples of different particle sizes (S1= 3-8 nm, S2= 4-8 nm and S3 = 5-9 nm) used in this study with three different concentrations 60, 77 and 26 µg/mL of Ag nanoparticles in the solutions respectively.
2.3. Bacterial Strains
Bacterial strains were isolated from water supply of different sectors of Islamabad and identified by API 20E method. These include Enterobacter cloaceae, Escherichia coli, Pseudomonas aeruginosa, Cronobacter sakazakii, Pantoea agglomeran, Serratia marcescens, Aeromonas hydrophila and Klebsiella pneumoniae.
2.4. Bioassay
Antibacterial activity of Ag nanoparticles was tested in triplicate by using agar well diffusion method (Perez et al., 1990). 1.5 × 108 Colony Forming Units (CFU/ml) of each microorganismwas inoculated on Muller Hinton (MH) agar. Working volume 120 µL of different Ag nanoparticle sample was used with 100 µL of distilled water as control. Diameter of zones of inhibition was noted after 24 hrs of incubation at 37 °C.
3. Results
Antibacterial activity of Ag nanoparticles (Ag NPs) was tested against different Gram negative waterborne bacterial strains which were isolated from water supply of Islamabad working under Capital Development Authority (CDA). Casein stabilized (S1) and Citrate stabilized (S2) silver nanoparticles showed activity against various water borne bacterial strains. To find out the effect of different surface ligands of Ag NPs on bacteria, the concentration difference was eliminated by normalizing the results to the particle concentration. Casein stabilized particles (S1) were more effective than citrate stabilized particles (S2) against all the tested bacterial strains as shown in Figure 2.
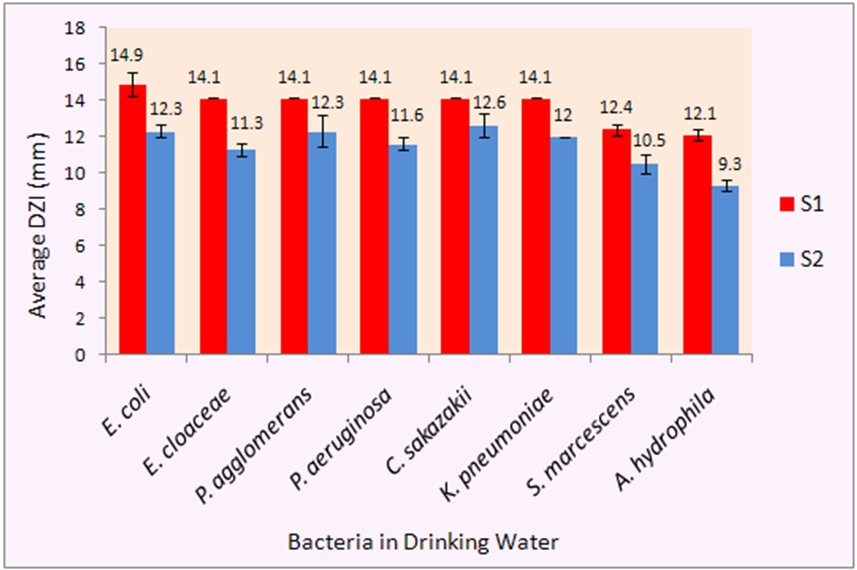
Antibacterial activity of S1 and S2 varied from 9.3 to 14.9 mm for different bacterial strains. Previously different studies were carried out on antibacterial activity of Ag NPs (Savithramma et al., 2011; Ruparelia et al., 2008) but due to different particles size, method of preparation and initial bacterial concentration used, direct comparison is difficult. S2 showed 12.3 mm inhibition zone against E. coli and 12.6 mm against C. sakazakii. Theivasanthi and Alagar (2011) also studied the effect of silver nanoparticles (24 nm particle size) against E. coli and reported 12 mm zones of inhibition. Furthermore, S2 showed 12 mm and 11.6 mm inhibition zones against K. pneumoniae and P. aeruginosa respectively. Prasad et al. (2011) reported a 6 mm zone of inhibition against K. pneumoniae when they used silver nanoparticles of 59 nm produced phytogenically. Another antibacterial study of silver nanoparticles produced from three different medicinal plants showed 12, 7 and 12 mm zones of inhibition against Klebsiella and 9, 6, 15 mm inhibition zones against Pseudomonas (Savithramma et al., 2011). The variation in results is most likely due to different particle sizes and methods used for synthesis of silver nanoparticles. S1 particles showed better activity than S2 against all the tested strains of bacteria.
E. coli was most effectively inhibited with 14.9 mm zone of inhibition and A. hydrophila was observed to be the most resistant against silver nanoparticles among the eight Gram negative bacteria tested. S3 showed no activity. Our results have shown good relevance with the previous literature although direct comparison is difficult due to differences of parameters regarding the use of initial bacterial count and amount of Ag NPs tested.
4. Conclusion
We evaluated the effect of surface stabilizers of silver nanoparticles between the range of 3-10 nm on the waterborne bacterial strains. Citrate stabilized silver nanoparticles and Casein stabilized silver nanoparticles showed antibacterial activity against all the tested strains. The biocidal action of citrate stabilized particles against multidrug resistant bacteria is known (Borah et al., 2012). C. sakazakii (12.6 mm) was most sensitive to citrate stabilized particles, followed by E. coli (12.3 mm), K. pneumoniae (12 mm) and P. aeruginosa (11.6 mm). Casein stabilized particles (Sample 1) have shown marked antibacterial effect as compared to citrate stabilized particles. Therefore, it can be concluded that casein is a better surface ligand in terms of antibacterial effect as compared to citrate, E. coli being the most effectively inhibited with 14.9 mm zone of inhibition.
Enterobacter cloaceae, Pantoea agglomerans and Cronobacter sakazakii were tested for the first time to our knowledge. A detailed study is, however, required for the production of silver nanoparticles with better surface stabilizers for improved antibacterial activity. Further, the effect of the shape of the Ag nanoparticles against the bacterial strains used is under investigation. Polymer stabilized silver nanoparticles (S3) showed no activity against any strain tested. This might be due to low concentration (26 µg/mL) of silver nanoparticles in the sample. Other similar studies reported the antibacterial activity of silver nanoparticles. Bankura et al. (2012) studied the antimicrobial activity of dextran stabilized silver nanoparticles in aqueous medium and they reported the significant reduction of bacteria, Bacillus subtilis, Bacillus cereus, Escherichia coli, Staphylococcus aureus and Pseudomonas aeruginosa. Another study by Ashraf et al. (2012) reported the antibacterial activity of Polyhexamethylene biguanide (PHMB) functionalized silver nanoparticles against E. coli.
Further investigations are under process and we plan to study Ag nanoparticle incorporated clay filters which are cheap and commercially affordable for low income group people for the provision of clean drinking water, particularly in rural areas. We believe that this study is important for the public, particularly in the developing countries and also for others working in the area of antibacterial effects of nanoparticles in drinking water.
Acknowledgements
The authors gratefully acknowledge the funding provided by Pakistan Academy of Sciences (PAS) for this research project and the support and encouragement of Dr. Abdul Basit, the Chancellor of the Preston University. The cooperation of the Water Management Wing of CDA is gratefully acknoweldged. We are grateful to the management of the PINSTECH laboratories for providing help in the measurements of the concentration of Ag nanoparticles in the samples used. We gratefully acknowledge the suggestions of Professor M. Fayyaz Chaudhary in the paper.
References
Ashraf, S., Akhtar, A., Ghauri, M. A., Rajoka, M. I., Khalid, Z. M., Hussain, I. (2012). Polyhexamethylene biguanide functionalized cationic silver nanoparticles for enhanced antimicrobial activity. Nanoscale Research Letters View Article
Ashraf, S., Abbasi, A. Z., Pfeiffer, C., Hussain S. Z., Khalid, Z. M., Gil, P. R., Parak, W. J., Hussain I. (2013). Protein-mediated synthesis, pH-induced reversible agglomeration and cellular interaction of silver nanoparticles toxicity. Colloids and Surfaces B: Biointerfaces. 102, 511– 518. View Article
Badireddy, A. R., Hotze, E. M., Chellam, S., Alvarez, P. J. J., Wiesner M.R. (2007). Inactivation of bacteriophages via photosensitization of fullerol nanoparticles. Environmental Science and Technology, 41, 6627-6632. View Article
Baker, C., Pradhan, A., Pakstis, L., Pochan, D. J., Shah, S. I. (2005). Synthesis and antibacterial properties of silver nanoparticles. Journal of Nanoscience and Nanotechnology 5, 244–249. View Article
Bankura, K. P., Maity, D., Mollick, M. M., Mondal, D., Bhowmick, B., Chakraborty, A., Sarkar, J., Acharya, K., Chattopadhyay, D., Bain, M. K. (2012). Synthesis, characterization and antimicrobial activity of dextran stabilized silver nanoparticles in aqueous medium. Carbohydrate Polymers, 89, 1159–1165. View Article
Borah, D., Buragohain, P., Saikia, A., Yadav, R. N. S. (2012). Synthesis and Evaluation of Antimicrobial Silver Nanoparticles on Multidrug-Resistant Bacterial Isolates from Urine Samples of Diabetic Patients and Infected Human Soft Tissues. Bionanosci., 4, 322-328.
Chamundeeswari, M., Sobhana, S. S., Jacob, J. P., Kumar, M. G., Devi, M. P., Sastry, T. P., Mandal, A. B. (2010). Preparation, characterization and evaluation of a biopolymeric gold nanocomposite with antimicrobial activity. Biotechnology Applied Biochemistry, 55, 29–35. View Article
Elchiguerra, J. L., Burt, J. L., Morones, J. R., Camacho-Bragado, A., Gao, X., Lara, H. H., Yacaman, M. J. (2005). Interaction of silver nanoparticles with HIV-1. Journal of Nanobiotechnology, 3:6. View Article
Huang, W. C., Tsai, P. J., Chen, Y. C. (2009). Multifunctional Fe3O4@Au nanoeggs as photothermal agents for selective killing of nosocomial and antibiotic-resistant bacteria. Small, 5(1), 51–56. View Article
Kang, S., Pinault, M., Pfefferle, L. D., Elimelech, M. (2007). Single walled carbon nanotubes exhibit strong antimicrobial activity. Langmuir, 23, 8670-8673. View Article
Khaydarov, R. R., Khaydarov, R. A., Gapurova, O., Estrin, Y., Evgrafova, S., Scheper, T, Cho, S. Y. (2009). "Antimicrobial effects of silver nanoparticles synthesized by an electrochemical method" in Book "Nanostructured material for Advanced Technological Applications", NATO Science for Peace and Security Series B: Physics and Biophysics, Springer, Part 5, Netherlands, pp. 215-218. View Article
Kim, J. S., Kuk, E., Yu, K. M., Kim, J. H., Park, S. J., Lee, H. J., Kim, S. H., Park, Y. K., Hwang, C. Y., Kim, Y. K., Lee, Y. S., Jeong, D. H., Cho, M. H. (2007). Antimicrobial effects of silver nanoparticles. Nanomedicine: Nanotechnology Biology and Medicine. 3(1), 95-101. View Article
Klasen, H. J. (2000). Historical review of the use of silver in the treatment of burns. I. early uses. Burns, 26, 117–130. View Article
Li, W.-R., Xie, X.-B., Shi, Q.-S., Zeng, H.-Y., Ou-Yang, Y.-S., Chen, Y.-B. (2010). Antibacterial activity and mechanism of silver nanoparticles on Escherichia coli. Applied Microbiology and Biotechnology, 85(4), 1115-1122. View Article
Liu Y., He L., Mustapha A., Li H., Hu Z.Q., Lin M. (2009). Antibacterial activities of zinc oxide nanoparticles against Escherichia coli O157:H7. Journal of Applied Microbiology, 107, 1193–1201. View Article
Madigan, M., Martinko, J. (2005). Brock biology of Microorganisms 11th edition, Prentice Hall Publishers, Englewood Cliffs.
Morones, J. R., Elechiguerra, J. L., Camacho, A., Holt, K., Kouri, J. B., Ramirez, J. T., Yacaman, M. J. (2005). The bactericidal effect of silver nanoparticles. Nanotechnology, 16(10), 2346-2353. View Article
Pal, S., Tak, Y. K., Song, J. M. (2007). Does the antibacterial activity of silver nanoparticles depend on the shape of nanoparticle? A study of Gram-negative bacterium E. coli Applied and Environmental Microbiology, 73(6), 1712–1720. View Article
Panacek, A., Kvitech, L., Prucek, R., Kolar, M., Vecerova, R., Pizrova, N., Sharma, V. K., Nevecna, T., Zboril, R. (2006). Silver colloid nanoparticles: Synthesis, characterization and their antibacterial activity. Journal of Physical Chemistry B., 110, 16248-16253. View Article
Parameswari, E., Udayasoorian, C., Sebastian, S. P., Jayabalakrishnan, R. M. (2010). The bactericidal potential of silver nanoparticles. International Research Journal of Biotechnology, 1(3), 44-49. View Article
Perez, C., Pauli, M., Bazerque, P. (1990). An antibacterial assay by agar well diffusion method. Acta. Bio. Et. Med. Exp. 15, 113-115.
Prasad, T. N. V. K. V., Elumalai, E. K., Khateeja, S. (2011). Evaluation of the antimicrobial efficacy of phytogenic silver nanoparticles. Asian Pacific Journal of Tropical Biomedicine, 1(1), S82-S85. View Article
Raimondi, F., Scherer, G. G., Kotz, R., Wokaun, A. (2005). Nanoparticles in energy technology: examples from electrochemistry and catalysis. Angewandte Chemie International Edition, 44, 2190–2209. View Article
Ruparelia, J. P., Chatterjee, A. K., Duttagupta, S. P., Mukherji, S. (2008). Strain specificity in antimicrobial activity of silver and copper nanoparticles. Acta Biomaterialia, 4(3), 707-16. View Article
Savithramma, N., Linga Rao, M., Rukmini, K., Suvarnalatha, Devi, P. (2011). Antimicrobial activity of silver nanoparticles synthesized by using medicinal plants. International Journal ChemTech. Research, 3(3), 1394-1402. View Article
Sharma, V. K., Yngard, R. A., Liu, Y. (2008). Silver nanoparticles: green synthesis and their antimicrobial activities. Advances in Colloid and Interface Science, 145, 83–96. View Article
Shrivastava, S., Bera, T., Roy, A., Singh, G., Ramachandrarao, P., Dash, D. (2007). Characterization of enhanced antibacterial effects of novel silver nanoparticles. Nanotechnology, 18, 1-9. View Article
Silvestry-Rodriguez, N., Bright, K. R., Uhlmann, D. R., Slack, D. C., Gerba, C. P. (2007). Inactivation of Pseudomonas aeruginosa and Aeromonas hydrophila by silver in tap water. Journal of Environmental Science and Health Part A. 42, 1579–1584. View Article
Sondi, I., Salopek-Sondi, B. (2004). Silver nanoparticles as antimicrobial agent: a case study on E. coli as a model for Gram-negative bacteria. Journal of Colloid and Interface Science, 275, 177–182. View Article
Turkevich, J., Stevenson, P. C., Hillier, J. (1951). A study of the nucleation and growth processes in the synthesis of colloidal gold. Discussions of the Faraday Society, 11, 55-75. View Article
Tiwari, D. K., Behari, J. (2009). Biocidal nature of combined treatment of Ag nanoparticle and ultrasonic irradiation in Escherichia coli dh5". Advances in Biological Research, 3, 89-95. View Article