Volume 1, Issue 1, Year 2012 - Pages 1-8
DOI: 10.11159/ijtan.2012.001
Isoprostanes as Biomarkers for In Vivo Evaluation of Nanoparticle-induced Oxidative Stress: A Study with Silica Nanoparticles Doped with Cadmium
Teresa Coccini¹, Elisa Roda¹, Luigi Manzo,Maugeri¹, Sergio Barni², Cinzia Signorini³
Maugeri Foundation IRCCS Institute of Pavia and University of Pavia
Toxicology Division and European Centre for Nanomedicine
Via Maugeri 10, Pavia, Italy I-27100
teresa.coccini@fsm.it; elisa.roda@fsm.it; luigi.manzo@fsm.it
University of Pavia, Department of Biology and Biotechnology "L. Spallanzani"
Via Ferrata 9, Pavia, Italy I-27100
barni@unipv.it
University of Siena, Department of Pathophysiology, Experimental Medicine,
and Public Health
Via Aldo Moro, Siena, Italy I-53100
cinzia.signorini@unisi.it
Abstract - The application of silica/cadmium containing nanomaterials in medicine and technology has attracted much attention in the latest years. However, information on toxicity, exposure and health impact of these nanomaterials is still limited. In this study, in vivo effects of silica nanoparticles (SiNPs) doped with Cd (SiNPs-Cd, 1 mg/rat), soluble CdCl2 (400 mg/rat), or SiNPs (600 mg/rat) have been investigated by evaluating F2-isoprostanes (F2-IsoPs) and superoxide dismutase (SOD1) as markers of oxidative stress 24 hr, 7 and 30 days after intra-tracheal (i.t.) instillation to rats. Free and esterified F2-IsoPs were evaluated in lung and plasma samples by GC/NICI-MS/MS analysis, and SOD1 expression in pulmonary tissue by immunocytochemistry. The present paper also gives an overview of current scientific knowledge and application of F2-IsoPs as oxidative stress markers.
Thirty days after exposure, pulmonary total F2-IsoPs were increased by 56% and 43% in CdCl2 and SiNPs-Cd groups, respectively, compared to controls (32.8±7.8 ng/g). Parallel elevation of free F2-IsoPs was observed in plasma samples (by 113 % and 95% in CdCl2 and SiNPs-Cd groups, respectively), compared to controls (28±8 pg/ml). These effects were already detectable at day 7 and lasted until day 30 post-exposure. Pulmonary SOD1-immunoreactivity was significantly enhanced in a time-dependent manner (7 days <30 days) after both CdCl2 and SiNPs-Cd treatments with the latter causing more pronounced effects. SiNPs did not influence SOD1-immunolabeling.
The results indicate the (i) potential of engineered SiNPs-Cd to cause long-lasting oxidative tissue injury following pulmonary exposure in rat and (ii) a possible role for plasma F2-IsoPs measurement as a predictive indicator of nanoparticle-induced oxidative insult.
Keywords: In Vivo, Cadmium Nanoparticles, Nanotoxicology, Silica, Isoprostanes, Superoxide Dismutase, Rat.
© Copyright 2012 Authors -This is an Open Access article published under the Creative Commons Attribution License terms. Unrestricted use, distribution, and reproduction in any medium are permitted, provided the original work is properly cited.
1. Introduction
With the exponentially growing production of engineered nanoproducts, occupational respiratory exposure to nanoparticles is expected to increase, and many aspects related to the small size and biological effects of these agents have raised concerns about safety (Maynard et al., 2006). Toxic processes that may result from the interaction of natural or engineered nanoparticles with living systems are linked to their high surface–to–volume ratio and enhanced reactivity with the biological system. Nanoparticles can be taken up actively by certain cell types (e.g., macrophages) and initiate formation of reactive oxygen and nitrogen species (ROS/NOS). If over- or chronically produced, these species can lead to increased transcription of proinflammatory mediators via intracellular signalling pathways, including calcium and oxidative stress pathways with consequent tissue changes and DNA, protein and lipid damage (Stone et al., 2007, Simkó et al., 2011). Several studies in animals have indicated a range of toxic effects that may be induced by nanoparticles such as oxidative stress, inflammation, granuloma formation, chronic pulmonary disease, immune system disorders, as well as increased risk of tumor development, and changes that may promote cardiovascular or neurodegenerative diseases (Donaldson et al., 2001; Oberdörster et al., 2005; Borm et al., 2006; Stern and McNeil, 2008; Hubbs et al., 2011; Liou et al., 2012). With respect to oxidative stress, development of specific, reliable and non-invasive testing methods for measuring this endpoint in humans may offer a valuable biomarker and in addition a research tool to elucidate the pathological role of free radicals in vivo. A list of representative biomarkers already used in clinical practice to evaluate the oxidative stress and inflammation outcomes is presented in Table 1.
Table 1. Values of measured contact resistance
- Blood /plasma antioxidant enzyme activity: Gpx-1, SOD, MPO (*) (systemic inflammation) |
- Exhaled breath nitric oxide, serum Clara cell protein (pulmonary inflammation) |
- Urine 8-hydroxydeoxyguanosine, plasma 8-hydroxydeoxyguanosine (oxidative DNA damage) |
- Fibrinogen, ICAM-1, IL-6, C reactive protein (cardiovascular system alterations, pro-thrombotic risk) |
F2-isoprostanes (F2-IsoPs) are specific products of nonenzymatic lipid peroxidation. They include a series of prostaglandin F2-like compounds which are generated by free radical-catalyzed peroxidation of phospholipid-bound arachidonic acid, through a cyclooxygenase-independent pathway (Milatovic et al., 2011). Initially formed in situ on phospholipids (esterified F2-IsoPs), F2-IsoPs are released into the circulation as free F2-IsoPs and owing to their relatively low reactivity can be easily measured in biological samples (e.g. plasma, urine) as oxidative stress markers (Milne et al., 2005; Halliweel and Lee, 2010). F2-IsoPs levels are found to be significantly increased, both in organs and body fluids, in diverse human disease conditions which share increased oxidative stress as a common pathological feature (Janssen, 2008). Recent studies in humans have suggested that F2-IsoPs may represent a more accurate marker of oxidative stress compared with other available methods (Table 2).
Table 2. Characteristics of F2-Isoprostanes as biomarkers of oxidative stress related to injury in vivo
- Specific products of lipid peroxidation |
- Well-known pathophysiological role |
- Rapidly released into the blood compartment after their in situ generation from phospholipids |
- More stable than other lipid peroxidation products (e.g., lipoperoxides and aldehydes) |
- Production increased in several animal models of oxidant injury |
The main objective of this study was, thus, to evaluate the validity of the circulating F2-IsoPs as marker of oxidative stress (Milatovic et al., 2011) associated to in vivo NPs exposure using a NP model namely silica nanoparticle (SiNPs) doped with cadmium (SiNPs-Cd). The response to SiNPs-Cd in terms of capacity to generate free radicals and induce oxidative stress was assessed in intratracheally (i.t.) treated rats by measuring lung and plasma levels of F2-IsoPs, as marker of oxidative stress, and immunocytochemical analysis of pulmonary copper-zinc superoxide dismutase (Cu/Zn-SOD1 or SOD1), an antioxidant isozyme also involved in oxidative stress pathway. The effect of SiNPs-Cd was evaluated at 24 hr, 7 and 30 days post-exposure in comparison with that caused by administration of equivalent amounts of CdCl2 and SiNPs.
Silica/cadmium containing nanomaterials are currently produced on an industrial scale for a variety of technological applications. Information on toxicity, exposure and health impact of these nanomaterials is still limited (Rzigalinski and Strobl, 2009; Vivero-Escoto et al., 2010). Dose-dependent cytotoxicity, increased reactive oxygen species levels and pro-inflammatory changes have been indicated by in vitro studies on SiNPs. A limited number of in vivo studies have demonstrated largely reversible lung inflammation, granuloma formation and focal emphysema, with no progressive lung fibrosis after respiratory exposure to these nanoparticles (Napierska et al., 2010). There is also a large body of evidence indicating that cadmium is toxic to the respiratory tract when inhaled (Oberdörster et al., 1994). Although the mechanisms of cadmium toxicity are not yet fully understood, several reports have described pulmonary inflammatory changes and induction of oxidative stress in response to cadmium inhalation exposure (IARC, 1993).
2. Materials and Methods
2. 1. Nanoparticles
The method used to produce SiNPs-Cd was described in a previous report (Coccini et al., 2012a). The particles presented amorphous and crystalline structure (confirmed by X-ray diffraction analysis), spherical form, primary particle size range of 20-80 nm and specific surface area of about 200 m2/g. Dynamic light scattering (DLS) determination of the SiNPs-Cd size distribution showed tendency to form aggregates and agglomerates of about 350 nm, and a zeta potential of – 23 mV (in deionized water). Flame-atomic absorption analysis was used to determine metal impurities and the release of cadmium from nanoparticles dispersed in physiological solution. Maximum cadmium release was 15% after 16 hr. Further metal release was negligible in the subsequent 10-day period. Main impurities were Ca (0.3%), Na (0.2%), K (0.2%), Fe (0.04%) and Mn (0.001%). Other metals were present in quantities less than 1 ‰. Cadmium and silica contents in the nanoparticles were 32.5% and 24.1%, respectively. CdCl2 was purchased from Sigma Aldrich (Milan, Italy) and SiNPs from Degussa GmbH (Germany).
2. 2. Animal Treatment
All experimental procedures were performed in compliance with the European Council Directive 86/609/EEC on the care and use of laboratory animals. Adult 12-weeks old male Sprague–Dawley rats (Charles River Italia, Calco, Italy) were allowed to acclimatize for at least 2 weeks before treatment, and kept in an artificial 12 h light:12 h dark cycle with humidity at 50±10% throughout the experiment. Animals were provided rat chow (4RF21 diet) and tap water ad libitum. Rats were anesthetized with pentobarbital sodium and treated with a single i.t. instillation of SiNPs-Cd (1 mg/rat, corresponding to about 250 µg Cd/rat). Separate groups of animals received i.t. an equivalent cadmium dose as CdCl2 (400 µg Cd/rat), SiNPs (600 µg/rat) or 0.1 ml/rat of saline (control). Treatment groups consisted of 6 animals/group at each time point. SiNPs-Cd suspension was vortexed on ice just before the exposure to force nanoparticle dispersion and avoid formation of agglomerates. No surfactants or solvents were used.
Biochemical and immunohistochemical evaluations were performed 24 hr, 7 and 30 days after treatment. At each time point, rats were anesthetized by i.p. injection of 35% chloral hydrate (100 µl/100 g b.w.) and from a set of animals (n=3) lungs were removed and blood collected in heparinized tubes for the isoprostane analyses. The blood samples were centrifuged at 2400 g for 15 min at 4 °C; the platelet-poor plasma was saved and the buffy coat was removed by aspiration. The remaining set of animals (n=3) was used to examine SOD in lung tissue by immunocytochemistry after vascular perfusion of fixative. The trachea was cannulated, and laparotomy was performed. The pulmonary artery was cannulated via the ventricle, and an outflow cannula was inserted into the left atrium. In quick succession, the tracheal cannula was connected to about 7 cm H2O pressure source to inflate the lungs with air, and clearing solution (saline with 100 U/ml heparin, 350 mosM sucrose) was perfused via the pulmonary artery. After blood was cleared from the lungs, the perfusate was switched to fixative consisting of 4% paraformaldehyde in 0.1 M phosphate buffer (pH 7.4). After fixation, the lungs were carefully removed.
2. 3. Oxidative Stress Evaluation
2.3.1. F2-Isoprostanes (F2-IsoPs) in Plasma and Lung
Free and total (sum of free plus esterified) F2-IsoPs were determined by gas chromatography/tandem mass spectrometry (GC/NICI-MS/MS) in plasma and lung samples, respectively, as described by Signorini et al. (2003, 2009). In previous studies (Aldinucci et al., 2007; De Felice et al., 2009; Signorini et al., 2003, 2008, 2009; Soffler et al., 2010), GC/NICI-MS/MS has proved to be a reliable procedure (in term of specificity, repeatability and accuracy) to assess F2-IsoPs as indicators of free radical-induced lipid peroxidation.
Plasma free, and lung total F2-IsoPs were expressed as picograms per millilitre or nanograms per gram, respectively. The calibration curve correlations were adequate (r2 = 0.994 for free F2-IsoPs; and r2=0.9987 for total F2-IsoPs); accuracy was 97.8% (free F2-IsoPs ), and 98.5% (total F2-IsoPs); variability coefficient were 2.5, and 2.2%, for free and total F2-IsoPs, respectively. The minimum detection limit was 5 pg/ml.
2.3.2. SOD1 in Lung
2.3.2.1 Lung Sampling for Immunocytochemistry
The top and the bottom regions of the right lungs were dissected. Tissue samples were obtained according to a stratified random sampling scheme which is a recommended method to reduce the variability and compensate for the existing regional differences in lung tissue (Weibel, 1979). From each slice, 2-3 blocks were systematically derived, washed in NaCl 0.9% and post-fixed by immersion for 7 hr in 4% paraformaldehyde in 0.1 M phosphate buffer (pH 7.4), dehydrated through a graded series of ethanol and finally embedded in Paraplast. Eight micrometer thick sections of the samples were cut in the transversal plane and collected on silan-coated slides. To avoid possible staining differences due to small changes in the procedure, the reactions were carried out simultaneously on slides of control and treated animals at all stages.
2.3.2.2.Immunocytochemistry for SOD1
Immunocytochemistry was performed using commercial antibodies on rat lung specimens to localize presence and tissue distribution of the SOD1 isozyme, as a marker of inflammation-related oxidative stress. Lung sections of control and treated rats were incubated overnight at room temperature with the primary rabbit polyclonal antibody against SOD1 (Santa Cruz Biotechnology, Santa Cruz, CA, USA) diluted 1:100 in PBS. Biotinylated anti-rabbit secondary antibody and an avidin biotinylated horseradish peroxidase complex (Vector Laboratories, Burlingame, CA, USA) were used to reveal the sites of antigen/antibody interaction. The 3,3'-diaminobenzidine tetrahydrochloride peroxidase substrate (Sigma, St. Louis, MO, USA) was used as chromogen, and Haematoxylin was employed for nuclear counterstaining. Then, the sections were dehydrated in ethanol, cleared in xylene, and finally mounted in Eukitt (Kindler, Freiburg, Germany). As negative controls, some sections were incubated with phosphate-buffer saline in absence of the primary antibody. No immunoreactivity was observed in this condition.
2. 4. Statistical Analysis
Isoprostane data were presented as means standard deviation (SD) for normally distributed variables. Differences between groups were evaluated using independent-sample t test (continuous normally distributed data). Two-tailed P values of less than 0.05 were considered significant. In all graphs, error bars represent the standard deviation of the mean.
Differential immunolabeling expression data were not normally distributed; therefore, the Kruskal-Wallis nonparametric test was used. Statistical significance is indicated with a * (P value < 0.05).
3. Results
3.1. Lung and Plasma F2-Isoprostanes
In lungs (Figure 1), total F2-IsoPs levels were not modified at the earlier time points (24 hr and 7 days) after either treatments (CdCl2 or SiNPs-Cd), while significantly increased levels of F2-IsoPs were found after 30 days in both groups: F2-isoprostane enhancements were 56% and 43% in CdCl2 and SiNPs-Cd groups, respectively, compared to controls (32.8±7.8 ng/g).
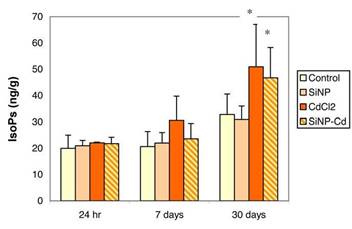
As shown in Figure 2, increased free F2-IsoPs levels were also observed in plasma samples. Changes in plasma F2-IsoPs were already observable at 7 days (by 94.9% and 79.5% in CdCl2 and SiNPs-Cd groups, respectively) and increases were still present at 30 days post-exposure (112.7% and 95.1% in CdCl2 and SiNPs-Cd groups, respectively, compared to control, 28 ± 8 pg/ml).
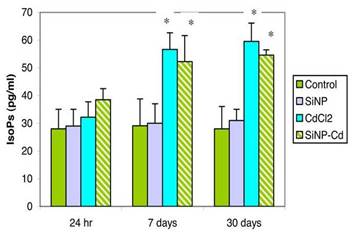
At both 7 and 30 days post exposure, the lung and plasma F2-IsoPs levels were not significantly different between CdCl2 and SiNPs-Cd groups.
3.2. Pulmonary SOD1
SOD1-immunoreactivity was remarkably enhanced in a time-dependent manner in lung tissue samples after both CdCl2 and SiNPs-Cd, with a more pronounced SOD1 immunoreactivity occurring at day 30 post treatment, especially in the animals given SiNPs-Cd (Figure 3). Administration of SiNPs caused no change in SOD1-immunolabeling compared to control rats (Table 3). Noticeably, numerous immunopositive activated macrophages were detected in the bronchiolar and alveolar epithelium of treated animals, particularly evident in collapsed areas, appearing heavily labelled. The localization and distribution of (Cu/Zn-SOD) SOD1, revealed an extensive spreading in bronchiolar and alveolar cells, evidencing the cellular response to injury, which was more intense for SiNPs-Cd observable starting at 7 days after i.t. exposure (Table 3).
Table 3. Expression of immunolabelling for SOD1 on a semiquantitative evaluation.
SOD1 |
||||
|
24 h |
7 days |
30 days |
p Value |
Control |
± |
± |
± |
|
SiNP |
± |
+ |
+± |
NS |
CdCl2 |
± |
+± |
++ |
* |
SiNP-Cd |
± |
++ |
++± |
* |
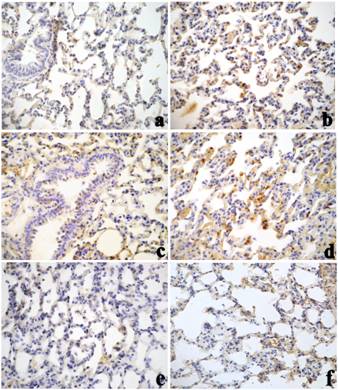
Cadmium exposure has been shown to activate cell defence processes, such as metallothionein synthesis, increase in cellular glutathione levels, and activation of antioxidant transcription factor Nrf2 (Liu et al., 2009). SOD1 over-expression may thus represents an additional adaptive defence mechanism that is activated by cells in response to Cd-induced prooxidative insult.
4. Discussion
Many disease conditions are characterized by ROS accumulation and "oxidative stress" which are thought to contribute to the pathogenesis of a number of human diseases including lung pathology (Thannickal and Fanburg, 2000; Janssen, 2008). ROS producing lipid peroxidation and DNA damage have been implicated in cadmium toxicity (Liu et al., 2009). Recent investigations in rats exposed to cadmium indicated Cd-induced lipid peroxidation in several organs (Galazyn-Sidorczuk et al., 2011) and dose-dependent changes in several oxidative stress markers including F2-IsoPs (Rogalska et al., 2009).
In our study, oxidative stress was observed in lung tissues and plasma after both CdCl2 and SiNPs-Cd treatments. The toxic response was reflected by elevated levels of F2-IsoPs and pulmonary over-expression of SOD1. In SiNPs-Cd-treated animals, the pattern of changes in isoprostane levels was organ- and time-dependent: in lung, changes were not apparent until 4 weeks after dosing; in plasma, F2-IsoPs levels were already increased at day 7 and were still enhanced 30 days after treatment. Similarly, elevated plasma F2-IsoPs levels were found in rats 7 and 30 days after i.t. instillation of CdCl2. These results indicated comparable oxidative stress response in lung tissues after administration of cadmium as inorganic metal or Cd-nanoparticles. Pulmonary changes were delayed in onset and were preceded by marked increase in F2-IsoPs levels in plasma suggesting that peripheral plasma may be a sensitive target for Cd-induced lipid peroxidation and that plasma F2-IsoPs may be early predictive indicators of later pulmonary oxidative insult.
In clinical practice, F2-IsoPs measurements in plasma or urine have recently been applied as biomarkers to assess the severity of respiratory disorders including acute lung injury, chronic obstructive pulmonary disease, allergic asthma, adult respiratory distress syndrome, pulmonary arterial hypertension, interstitial lung disease, and cystic fibrosis. These biomarkers have also been investigated in non-respiratory disease states such as alcoholic liver disease, hepatorenal syndrome, acute cholestasis, ischemia/reperfusion injury, and diabetes (Basu et al., 2008; Comporti et al., 2008; Janssen, 2008; Halliwell and Lee, 2010). Notably, elevated F2-IsoPs levels were reported in individuals exposed to environmental respiratory toxicants such as ozone, cigarette smoke, and allergens (Comporti et al., 2008; Janssen, 2008). In addition to their importance as indicator of oxidative damage, F2-IsoPs can also exert intrinsic biological effects by interacting with tissue receptors involved in constriction of pulmonary vessels and airways (Kang et al., 1993; Comporti et al., 2008). F2-IsoPs have been shown to exert biological action on platelets inducing shape changes thus altering the formation of thromboxane or irreversible aggregation in response to platelet agonist (Ting and Khasawneh, 2010; Milne et al., 2011). They can also cause vasoconstriction of renal arterioles, stimulation of DNA synthesis and cell proliferation on muscle vascular cells (Fukunaga, 1993) and endothelial cells (Yura, 1999). F2-IsoPs seems also to mediate the increased production of transforming growth factor-β1 (TGF-β1) in kidney mesangial and glomerular cells exposed to high ambient glucose such as that produced by streptozotocin-induced diabetes (Montero, 2000).
It is possible that the inflammatory effects observed in the animals treated with the nanomaterials could at least in part be mediated by release of F2-IsoPs secondary to oxidative stress. Presently, little is known about the time-course of lung tissue isoprostane accumulation in disease states and the correlation between changes in isoprostane levels and the onset/progression/regression of specific symptoms (Lakshminrusimha et al., 2006; Janssen, 2008).
Oxidative stress and inflammatory injury to airways are distinctive processes associated with high-level exposure to nanoparticles (Stone et al., 2007; Li et al., 2008; Simkó et al., 2011; Shvedova et al., 2012). In some instances, the primary respiratory effect of inhaled nanoparticles was shown to extend to extra-pulmonary sites (i.e., blood, vascular endothelium, secondary target organs) due to migration of the nanoparticles from lung to the systemic circulation or to secondary organ changes caused by circulating inflammatory factors (e.g., IL-6, IP-10 and TGF-b1) released from lung following local insult. Extra-pulmonary organ toxicity (e.g., nephrotoxicity) caused by inhaled nanoparticles have been documented in our previous studies on SiNPs-Cd (Coccini et al., 2011) and in experiments using carbon nanotubes (Reddy et al., 2010).
Additional issues considered in this study were those regarding the toxic potential of Cd-containing NPs compared to Cd salt and the effect of silica nanoparticles absorbed alone. Comparable oxidative stress responses occurred after administration of CdCl2 or Cd-nanoparticles. In lung and plasma, F2-IsoPs levels were similarly modulated in the two types of treatment, and the pulmonary expression of SOD1, an enzyme physiologically involved in oxidative stress protection, was also similarly increased in both SiNPs-Cd and CdCl2 groups. The SOD1changes were seen at the day 7 and were still observable 30 days post-exposure. On the other hand, toxicogenomic studies on oxidative stress pathways indicated remarkable differences in the gene expression profiles in rat lung and kidney after administration of SiNPs-Cd or equivalent amount of CdCl2 (Coccini et al., 2011, 2012b).
SiNPs are generally considered to be non-toxic and are largely investigated as potential drug delivery systems (Mamaeva et al., 2012). However, experiments in cell cultures or animal models have indicated dose-dependent cytotoxicity, increased reactive oxygen species, and reversible lung inflammation after exposure to SiNPs (Park and Park, 2009; Napierska et al., 2010; Panas et al., 2012). In the present study, the response to SiNPs differed from that observed with SiNPs-Cd as the latter but not SiNPs were effective in modifying pulmonary SOD1 expression and tissue levels of isoprostanes.
In summary, delayed occurrence of pulmonary oxidative stress was observed after i.t. instillation of SiNPs-Cd in rats. The effects SiNPs-Cd on tissue isoprostanes and pulmonary SOD1 were comparable to the effects caused by CdCl2. No changes involving these markers were observed in animals treated with SiNPs. These findings would suggest a primary role of the cadmium moiety in the biological response to SiNPs-Cd. However, it seems unlikely that the changes produced by SiNPs-Cd merely reflected the action of cadmium ions released from nanoparticles. Preliminary chemical assays with SiNPs-Cd have indicated limited release of cadmium ions from the nanoparticles dispersed in physiological solution, the maximum metal release being ca. 15% over a 10-day period. Gene modulated by SiNPs-Cd were different than those modulated by CdCl2 at 30 days. The overall genomic data showed only partially overlapping with CdCl2 (Coccini et al., 2011). DLS experiments with the nanoparticles dispersed in physiological solution showed agglomeration and aggregation of SiNPs-Cd to extent greater than that found with SiNPs. The more pronounced tendency of SiNPs-Cd to form aggregates and agglomerates may offer an additional mechanistic explanation of our experimental findings.
The results provided an example on how measurements of F2-IsoPs levels in plasma may be valuable as a predictive indicator of NP-induced oxidative lung damage. Further studies are needed to establish the validity of F2-IsoPs measurements in field studies such as biomonitoring exposure to engineered nanomaterials in worksites and human safety assessment of nanomedicines.
Acknowledgements
Work was supported by Grants from European Commission, Italian Ministries of Health, Research and Education, and CARIPLO Foundation (rif. 2011-2096). The authors wish to acknowledge Mr. Davide Acerbi for his excellent technical assistance.
References
Aldinucci, C., Carretta, A., Ciccoli, L., Leoncini, S., Signorini, C., Buonocore, G., Pessina, G.P. (2007). Hypoxia affects the physiological behavior of rat cortical synaptosomes. Free Radical Biology and Medicine, 42, 1749-1756.View Article
Basu, S. (2008). Comprehensive invited review F2-Isoprostanes in human health and diseases: from molecular mechanisms to clinical implications. Antioxidants and Redox Signaling, 10, 1405-1434. View Article
Borm, P. J., Robbins, D., Haubold, S., Kuhlbusch, T., Fissan, H., Donaldson, K., Schins, R., Stone, V., Kreyling, W., Lademann, J., Krutmann, J., Warheit, D., Oberdörster, E. (2006). The potential risks of nanomaterials: a review carried out for ECETOC. Particle and Fibre Toxicoloy, 3, 11-45. View Article
Coccini T., Fabbri M., Roda E., Sacco M. G., Manzo L., Gribaldo L. (2011). Gene expression analysis in rat lung after intratracheal exposure to nanoparticles doped with cadmium. Journal of Physics: Conference Series, 304. View Article
Coccini, T., Barni, S., Vaccarone, R., Mustarelli, P., Manzo, L., Roda, E. (2012a). Pulmonary Toxicity of Instilled Cadmium-Doped Silica Nanoparticles During Acute and Subacute stages in Rats. Histology and Histopathology, in press. View Article
Coccini, T., Roda, E., Fabbri, M., Sacco, M. G., Gribaldo, L., Manzo, L. (2012b). Gene expression profiling in rat kidney after intra-tracheal exposure to cadmium-doped nanoparticles. Journal of Nanoparticle Research, 14, 925. View Article
Comporti, M., Signorini, C., Arezzini, B., Vecchio, D., Monaco, B., Gardi, C. (2008). F2-isoprostanes are not just markers of oxidative stress. Free Radical Biology and Medicine., 44, 247-256.View Article
De Felice, C., Ciccoli, L., Leoncini, S., Signorini, C., Rossi, M., Vannuccini, L., Guazzi, G., Latini, G., Comporti, M., Valacchi, G., Hayek, J. (2009). Systemic oxidative stress in classic Rett syndrome. Free Radical Biology Medical, 47, 440-448. View Article
Donaldson, K., Stone, V., Seaton, A., MacNee, W. (2001). Ambient particle inhalation and the cardiovascular system: potential mechanisms. Environmental Health Perspectives, 109, 523-527. View Article
Fukunaga, M., Makita, N., Roberts, L. J., Morrow, J. D., Takahashi, K., Badr, K. F. (1993). Evidence for the existence of F2-isoprostane receptors on rat vascular smooth muscle cells. American Journal of Physiology, 264, C1619–C1624. View Article
Galażyn-Sidorczuk, M., Brzóska, M. M., Rogalska, J., Roszczenko, A., Jurczuk, M. (2011). Effect of zinc supplementation on glutathione peroxidase activity and selenium concentration in the serum, liver and kidney of rats chronically exposed to cadmium. Journal of Trace Elements in Medicine and Biology., Epub ahead of print. View Article
Halliwell, B., Lee, C. Y. J. (2010). Using isoprostanes as biomarkers of oxidative stress: some rarely considered issues. Antioxidants and Redox Signaling, 13, 145-156. View Article
Hubbs, A. F., Mercer, R. R., Benkovic, S. A., Harkema, J., Sriram, K., Schwegler-Berry, D., Goravanahally, M. P., Nurkiewicz, T. R., Castranova, V., Sargent, L. M. (2011). Nanotoxicology-a pathologist's perspective. Toxicologic Pathology, 39, 301-324. View Article
IARC (International Agency for Research on Cancer) (1993) Cadmium and cadmium compounds. IARC Monogr. Eval. Carcinog. Risk Hum., 58, 119-237. View Article
Janssen, L. J. (2008). Isoprostanes and lung vascular pathology. American Journal of Respiratory Cell and Molecular Biology, 39, 383-389. View Article
Kang, K. H., Morrow, J. D., Roberts, L. J., Newman, J. H., Banerjee, M. (1993). Airway and vascular effects of 8-epi-prostaglandin F2 alpha in isolated perfused rat lung. Journal of Applied Physiology, 74, 460-465. View Article
Lakshminrusimha, S., Russell, J. A., Wedgwood, S., Gugino, S. F., Kazzaz, J. A., Davis, J. M., Steinhorn, R. H. (2006). Superoxide dismutase improves oxygenation and reduces oxidation in neonatal pulmonary hypertension. American Journal of Respiratory and Critical Care Medicine, 174, 1370-1377. View Article
Li, N., Xia, T., Nel, A. E. (2008). The role of oxidative stress in ambient particulate matter-induced lung diseases and its implications in the toxicity of engineered nanoparticles. Free Radical Biology and Medicine., 44, 1689-1699. View Article
Liu, J., Qu, W., Kadiiska, M.,B. (2009). Role of oxidative stress in cadmium toxicity and carcinogenesis. Toxicology and Applied Pharmacology, 238, 209-214. View Article
Liou, S-H., Tsou, T-C., Wang, S-L., Li, L-A., Chiang, H-C., Li, W-F., Lin, P-P., Lai, C-H., Lee, H-L., Lin, M-H., Hsu, J-H., Chen, C-R., Shih, T-S., Liao, H-Y., Chung, Y-T. (2012). Epidemiological study of health hazards among workers handling engineered nanomaterials. Journal of Nanoparticle Research, 14, 878. View Article
Mamaeva, V., Sahlgren, C., Lindén, M. (2012). Mesoporous silica nanoparticles in medicine-Recent advances. Advanced Drug Delivery Reviews. View Article
Maynard, A. D., Aitken, R. J., Butz, T., Colvin, V., Donaldson, K., Oberdörster, G., Philbert, M. A., Ryan, J., Seaton, A., Stone, V., Tinkle S. S., Tran, L., Walker, N. J., Warheit, D. B. (2006). Safe handling of nanotechnology. Nature, 444, 267-269. View Article
Milatovic, D., Montine, T. J., Aschner, M. (2011). Measurement of isoprostanes as markers of oxidative stress. Methods in Molecular Biology, 75, 195-204. View Article
Milne, G. L., Musiek, E. S., Morrow, J. D. (2005). F2-isoprostanes as markers of oxidative stress in vivo: an overview. Biomarkers, 10 Suppl 1, S10-S23. View Article
Milne, G. L., Yin, H., Hardy, K. D., Davies, S. S., Roberts II, L.,J. (2011). Isoprostane generation and function. Chemical Reviews, 111, 5973-5996. View Article
Montero, A., Munger K.A., Khan R.Z., Valdivielso J. M., Morrow J.D., Guasch A., Ziyadeh F.N., Badr K. F. (2000). F(2)-isoprostanes mediate high glucose-induced TGF-beta synthesis and glomerular proteinuria in experimental type I diabetes. Kidney International, 58, 1963–1972. View Article
Napierska, D., Thomassen, L. C. J., Lison, D., Martens, J. A., Hoet, P. H. (2010). The nanosilica hazard: another variable entity. Particle and Fibra Toxicology, 7, 39. View Article
Oberdörster, G., Cherian, M. G., Baggs, R. B. (1994). Correlation between cadmium-induced pulmonary carcinogenicity, metallothionein expression, and inflammatory processes: a species comparison. Environmental Health Perspectives, 102 Suppl 3, 257-263. View Article
Oberdörster, G., Oberdörster, E., Oberdörster, J. (2005). Nanotoxicology: an emerging discipline evolving from studies of ultrafine particles. Environmental Health Perspectives, 113, 823-839. View Article
Panas, A., Marquardt, C., Nalcaci, O., Bockhorn, W., Baumann, W., Paur, H. R., Mulhopt, S., Diabaté, S., Weiss, C. (2012). Screening of different metal oxide nanoparticles reveals selective toxicity and inflammatory potential of silica nanoparticles in lung epithelial cells and macrophages. Nanotoxicology, Epub ahead of print, 1-15. View Article
Park, E. J., Park, K. (2009). Oxidative stress and pro-inflammatory responses induced by silica nanoparticles in vivo and in vitro. Toxicology Letters, 184, 18-25. View Article
Reddy, A. R. N., Krishna, D. R., Reddy, Y. N., Himabindu, V. (2010). Translocation and extra pulmonary toxicities of multi wall carbon nanotubes in rats. Toxicology Mechanisms and Methods, 20, 267-272. View Article
Rogalska, J., Brzóska, M. M., Roszczenko, A., Moniuszko-Jakoniuk, J. (2009). Enhanced zinc consumption prevents cadmium-induced alterations in lipid metabolism in male rats. Chemico-Biological Interations, 177, 142-152. View Article
Rogalska, J., Brzóska, M. M., Roszczenko, A., Moniuszko-Jakoniuk, J. (2009). Enhanced zinc consumption prevents cadmium-induced alterations in lipid metabolism in male rats. Chemico-Biological Interations, 177, 142-152. View Article
Signorini, C., Comporti, M., Giorgi, G. (2003). Ion trap tandem mass spectrometric determination of F2-isoprostanes. Journal of Mass Spectrometry, 38, 1067-1074. View Article
Signorini, C., Ciccoli, L., Leoncini, S., Carloni, S., Perrone, S., Comporti M., Balduini W., Buonocore G. (2009). Free iron, total F2-isoprostanes and total F4-neuroprostanes in a model of neonatal hypoxic-ischemic encephalopathy: neuroprotective effect of melatonin. Journal of Pineal Research., 46, 148-154. View Article
Signorini, C., Perrone, S., Sgherri, C., Ciccoli, L., Buonocore, G., Leoncini, S., Rossi, V., Vecchio, D., Comporti, M. (2008). Plasma esterified F2-isoprostanes and oxidative stress in newborns: role of nonprotein-bound iron. Pediatric Research, 63, 287-291. View Article
Simkó, M., Gazsó, A., Fiedeler, U., Nentwich, M. (2011). Nanoparticles, free radicals and oxidative stress. NanoTrust-Dossier No. 012en, January 2011. View Article
Soffler, C., Campbell, V. L., Hassel, D. M. (2010). Measurement of urinary F2-isoprostanes as markers of in vivo lipid peroxidation: a comparison of enzyme immunoassays with gas chromatography-mass spectrometry in domestic animal species. Journal of Veterinary Diagnostic Investigation, 22, 200-209. View Article
Shvedova, A. A., Pietroiusti, A., Fadeel, B., Kagan, V. E. (2012). Mechanisms of carbon nanotube-induced toxicity: Focus on oxidative stress. Toxicology and Applied Pharmacology, 261, 121-133. View Article
Stern, S. T., McNeil, S. E. (2008). Nanotechnology safety concerns revisited. Toxicology Science, 101, 4-21. View Article
Stone, V., Johnston, H., Clift, M. J. (2007). Air pollution, ultrafine and nanoparticle toxicology: cellular and molecular interactions. IEEE Trans Nanobioscience, 6, 331–340. View Article
Thannickal, V. J., Fanburg, B. L. (2000). Reactive oxygen species in cell signalling. American Journal of Physiology - Lung Cellular and Molecular Physiology, 279, L1005–L1028. View Article
Ting, H. J., Khasawneh, F. T. (2010). Platelet function and Isoprostane biology. Should isoprostanes be the newest member of the orphan-ligand family? Journal of Biomedical Science, 17, 24. View Article
Vivero-Escoto, J. L., Slowing, I. I., Trewyn, B. G., Lin, V. S. (2010). Mesoporous silica nanoparticles for intracellular controlled drug delivery. Small, 6, 1952-1967. View Article
Yura, T., Fukunaga, M., Khan, R., Nassar, G. N., Badr, K. F., Montero A. (1999). Free-radical-generated F2-isoprostane stimulates cell proliferation and endothelin-1 expression on endothelial cells. Kidney International, 56, 471-478. View Article
Weibel, E. R. (1979). Stereological methods. Vol. 1: Practical methods for biological morphometry. Academic Press Inc. London. View Article